Bond order, a key concept in chemistry, quantifies the strength of a chemical bond between atoms in a molecule. It reflects the number of electron pairs shared between bonded atoms, providing insights into molecular stability and reactivity. For instance, double bonds, with two shared electron pairs, are typically stronger than single bonds with only one shared pair.
Understanding bond order is crucial for comprehending chemical bonding, molecular properties, and predicting reactivity. Its historical development, from Lewis’s electron-pair theory to molecular orbital theory, has deepened our understanding of chemical bonding.
This article delves into the methods for calculating bond order, exploring various approaches and their applications in chemistry.
How to Calculate Bond Order of Molecular
Bond order, a crucial concept in chemistry, quantifies the strength of chemical bonds in molecules. Understanding how to calculate bond order is essential for comprehending chemical bonding, molecular properties, and predicting reactivity.
- Electron Configuration
- Molecular Orbitals
- Resonance Structures
- Hybridization
- Delocalization
- Bond Length
- Bond Energy
- Magnetic Properties
- Spectroscopic Properties
These aspects are interconnected and provide insights into the nature of chemical bonds, their strength, and their impact on molecular behavior. By understanding how to calculate bond order, chemists can gain valuable information about the structure, reactivity, and properties of molecules.
Electron Configuration
Electron configuration, the distribution of electrons in atomic orbitals, plays a critical role in determining the bond order of molecules. The number of valence electrons, those in the outermost shell, dictates the bonding capacity of an atom. Atoms with unpaired valence electrons tend to form bonds to achieve a stable electron configuration, typically a filled valence shell with eight electrons (octet rule).
For instance, in a double bond between two carbon atoms, each carbon contributes two valence electrons, resulting in a total of four shared electrons and a bond order of two. This shared electron pair forms a covalent bond, increasing the stability of the molecule.
Understanding electron configuration is crucial for calculating bond order and predicting molecular properties. It enables chemists to determine the number of bonds an atom can form, the strength of those bonds, and the overall stability of the molecule.
Molecular Orbitals
Molecular orbitals, a cornerstone of quantum chemistry, are crucial for understanding and calculating bond order in molecules. They describe the wave-like behavior of electrons within a molecule, providing insights into electron distribution and bonding interactions.
- Atomic Orbitals: The foundation of molecular orbitals, atomic orbitals describe the electron distribution around individual atoms.
- Linear Combination of Atomic Orbitals (LCAO): Molecular orbitals result from the mathematical combination of atomic orbitals, leading to the formation of bonding and antibonding orbitals.
- Bonding Orbitals: Electrons in bonding orbitals contribute to the formation of chemical bonds by increasing electron density between nuclei.
- Antibonding Orbitals: Electrons in antibonding orbitals weaken or prevent bond formation by decreasing electron density between nuclei.
By analyzing molecular orbitals and their interactions, chemists can determine the number and strength of bonds in a molecule, providing valuable insights into molecular stability, reactivity, and properties.
Resonance Structures
Resonance structures are crucial for calculating bond order in molecules with delocalized electrons. They represent different electronic configurations of the same molecule, providing insights into electron distribution and bonding interactions.
- Equivalent Forms: Resonance structures are not different molecules but different representations of the same molecule, contributing to the overall resonance hybrid.
- Delocalized Electrons: Resonance structures arise when electrons are delocalized over multiple atoms, preventing their confinement to a single bond.
- Average Bond Order: Bond order in resonance structures is not a single value but an average of all contributing resonance structures.
- Benzene Example: The benzene ring is a classic example of resonance, with six delocalized electrons contributing to the aromatic stability of the molecule.
Understanding resonance structures enables chemists to calculate bond order accurately, considering the delocalization of electrons. It provides insights into molecular stability, reactivity, and various chemical phenomena.
Hybridization
In calculating bond order of molecules, hybridization, the concept of combining atomic orbitals to form new hybrid orbitals with different shapes and energies, plays a crucial role.
- sp3 Hybridization: When an atomic orbital hybridizes with three p orbitals, it forms four equivalent sp3 hybrid orbitals directed towards the corners of a tetrahedron.
- sp2 Hybridization: When an atomic orbital hybridizes with two p orbitals, it forms three equivalent sp2 hybrid orbitals lying in a plane and one unhybridized p orbital.
- sp Hybridization: When an atomic orbital hybridizes with one p orbital, it forms two equivalent sp hybrid orbitals and two unhybridized p orbitals.
- Examples: sp3 hybridization is observed in methane (CH4), sp2 hybridization in ethene (C2H4), and sp hybridization in acetylene (C2H2).
The type of hybridization affects the bond order and geometry of molecules. Hybridization provides a deeper understanding of molecular bonding and the properties of various compounds.
Delocalization
Delocalization, a crucial aspect of calculating bond order in molecules, involves the dispersal of electrons over multiple atoms rather than their confinement to specific bonds. This phenomenon affects bond order and molecular properties, offering valuable insights into chemical structure and reactivity.
- Resonance Structures: Delocalization often arises in resonance structures, where multiple electronic configurations contribute to the overall molecular structure. These structures depict the delocalization of electrons over several atoms, leading to an average bond order.
- Pi Bonds: Delocalized electrons are commonly found in pi bonds, which involve lateral overlap of p orbitals. The delocalization of pi electrons contributes to the stability and unique properties of compounds like benzene and other aromatic molecules.
- Metallic Bonding: In metals, valence electrons are delocalized throughout the crystal lattice, forming a “sea of electrons.” This delocalization results in high electrical and thermal conductivity characteristic of metallic materials.
- Allotropes: Delocalization can influence the formation of allotropes, different structural forms of an element. Carbon, for instance, exists as graphite and diamond due to the delocalization of electrons in the former and localized electrons in the latter.
Understanding delocalization is crucial for accurately calculating bond order and comprehending the behavior of molecules. It provides insights into molecular stability, reactivity, and various chemical phenomena, expanding our knowledge of molecular bonding and its implications in chemistry.
Bond Length
Bond length, a crucial aspect in calculating bond order, provides valuable insights into the nature and strength of chemical bonds. It refers to the equilibrium distance between the nuclei of bonded atoms and plays a significant role in determining molecular properties and reactivity.
- Atomic Radii: Bond length is influenced by the atomic radii of the bonded atoms. Larger atoms generally form longer bonds due to increased internuclear distance.
- Bond Order: Bond length is inversely related to bond order. Stronger bonds, with higher bond orders, are shorter in length due to increased electron density between the nuclei.
- Hybridization: The hybridization of atomic orbitals affects bond length. Orbitals with greater s-character (e.g., sp3) form shorter bonds compared to those with less s-character (e.g., sp).
- Electronegativity: Bond length is influenced by the electronegativity of the bonded atoms. Bonds between atoms with significant electronegativity differences tend to be shorter due to the attraction of electrons towards the more electronegative atom.
Understanding bond length is crucial for accurately calculating bond order and predicting molecular properties. It provides insights into bond strength, molecular geometry, and reactivity, enabling chemists to understand and manipulate chemical systems more effectively.
Bond Energy
Bond energy, a fundamental concept in chemistry, quantifies the strength of chemical bonds between atoms in a molecule. It represents the energy required to break a bond and is closely related to bond order, a measure of the number of electron pairs shared between bonded atoms.
Bond order directly influences bond energy. Generally, stronger bonds, with higher bond orders, have greater bond energies. This relationship arises from the increased electron density between bonded atoms in higher bond orders, leading to stronger attractive forces and, consequently, higher bond energies. For instance, a triple bond, with three shared electron pairs, has a higher bond energy than a double bond, which has two shared electron pairs, and a single bond, with only one shared electron pair.
Understanding the relationship between bond energy and bond order is crucial for various chemical applications. For example, in designing new materials, chemists aim to create substances with specific properties by manipulating bond strengths. By controlling the bond order and, consequently, the bond energy, they can tailor materials for desired applications, such as high-strength alloys or lightweight composites.
In summary, bond energy and bond order are inextricably linked, with bond order serving as a key determinant of bond strength. Understanding this relationship enables chemists to predict molecular stability, design new materials, and manipulate chemical reactions, leading to advancements in diverse fields such as materials science, drug discovery, and energy storage.
Magnetic Properties
Magnetic properties are closely related to the calculation of bond order in molecules. Bond order, a measure of the number of electron pairs shared between bonded atoms, influences the magnetic behavior of molecules. This connection arises from the fundamental principles of electron spin and its alignment in molecular orbitals.
Unpaired electrons in molecular orbitals contribute to magnetic properties. When electrons are paired within a molecular orbital, their spins cancel each other out, resulting in a diamagnetic molecule. Conversely, molecules with unpaired electrons exhibit paramagnetic behavior due to the presence of net spin. The number of unpaired electrons directly affects the strength of the magnetic properties.
Understanding the relationship between bond order and magnetic properties is crucial in various chemical applications. For instance, in the field of materials science, magnetic properties play a vital role in designing materials with specific magnetic functionalities. By manipulating bond order and electron configuration, chemists can tailor materials for applications in electronics, data storage, and spintronics.
In summary, magnetic properties are intricately connected to bond order calculation, providing insights into the electronic structure and magnetic behavior of molecules. This understanding has significant implications in materials science and other fields, enabling the development of advanced materials with tailored magnetic properties for various technological applications.
Spectroscopic Properties
Spectroscopic properties are a critical component of calculating bond order in molecules. They provide valuable insights into the electronic structure, bonding, and molecular geometry, allowing chemists to determine the number of electron pairs shared between bonded atoms.
Spectroscopic techniques, such as ultraviolet-visible (UV-Vis) spectroscopy and infrared (IR) spectroscopy, measure the absorption or emission of electromagnetic radiation by molecules. These techniques provide information about the energy levels and transitions of electrons within the molecule. By analyzing the spectra, chemists can identify the functional groups, determine the molecular structure, and calculate the bond order.
For example, in UV-Vis spectroscopy, the absorption of light corresponds to the excitation of electrons from the ground state to an excited state. The wavelength of the absorbed light is related to the energy difference between the two states, which is influenced by the bond order. Higher bond orders result in shorter wavelengths of absorption due to the stronger bonding interactions.
Understanding the relationship between spectroscopic properties and bond order has significant practical applications. It enables chemists to identify and characterize unknown compounds, study reaction mechanisms, and design new materials with tailored properties. Spectroscopic techniques are also used in various fields, including analytical chemistry, biochemistry, and materials science.
Frequently Asked Questions
This section addresses common questions and clarifies key aspects of calculating bond order in molecules.
Question 1: What is bond order, and why is it essential in chemistry?
Answer: Bond order describes the strength of a chemical bond and reflects the number of electron pairs shared between bonded atoms. It is crucial for understanding molecular structure, stability, and reactivity.
Question 2: How is bond order calculated using molecular orbitals?
Answer: Bond order can be calculated based on the molecular orbital theory by determining the number of bonding and antibonding electrons in the molecular orbital diagram.
Question 3: Can bond order be fractional in certain molecules?
Answer: Yes, resonance structures can lead to fractional bond orders, indicating delocalization of electrons and resonance between multiple contributing structures.
Question 4: How does hybridization affect bond order?
Answer: Hybridization influences bond order by changing the number and type of atomic orbitals involved in bonding, affecting the strength and directionality of the bonds.
Question 5: What is the relationship between bond order and bond length?
Answer: Bond order is inversely proportional to bond length. Stronger bonds with higher bond orders have shorter bond lengths due to increased electron density and stronger attractive forces.
Question 6: How does bond order influence molecular properties?
Answer: Bond order impacts various molecular properties, including stability, reactivity, bond length, vibrational frequency, and magnetic behavior.
These FAQs provide a concise overview of the fundamental concepts and applications of bond order calculations. Understanding bond order is essential for deciphering molecular structures and predicting their properties, which plays a vital role in diverse fields such as chemistry, materials science, and biochemistry.
In the next section, we will delve deeper into advanced methods for calculating bond order, exploring computational approaches and their applications in complex molecular systems.
Tips for Calculating Bond Order of Molecules
This section provides practical tips to assist you in accurately calculating bond order, a crucial aspect of understanding molecular structure and bonding.
Tip 1: Determine the Electron Configuration of the Atoms: The number of valence electrons determines the bonding capacity of atoms. Identify the valence electrons to assess the potential bond formation.
Tip 2: Construct Molecular Orbital Diagrams: Visualize the molecular orbitals formed by the combination of atomic orbitals. The number of bonding and antibonding electrons in the molecular orbital diagram indicates the bond order.
Tip 3: Consider Resonance Structures: In resonance structures, electrons are delocalized over multiple atoms. Calculate the average bond order by considering all contributing resonance structures.
Tip 4: Analyze Hybridization: Hybridization influences the geometry and strength of bonds. Determine the hybridization of atomic orbitals to understand the bond order.
Tip 5: Relate Bond Order to Bond Length and Energy: Bond order is inversely proportional to bond length and directly proportional to bond energy. Utilize these relationships to estimate bond order when experimental data is unavailable.
These tips provide a practical framework for calculating bond order, enabling you to confidently assess molecular bonding and properties.
In the next section, we will explore advanced computational methods for bond order calculations, extending our understanding to complex molecular systems.
Conclusion
Our exploration of “How to Calculate Bond Order of Molecules” has provided valuable insights into the fundamental aspects of molecular bonding. We have examined the relationship between bond order and electron configuration, molecular orbitals, resonance structures, hybridization, and various molecular properties such as bond length, bond energy, and magnetic behavior.
Key points to remember include:
- Bond order is a measure of the strength of a chemical bond, reflecting the number of electron pairs shared between bonded atoms.
- Various methods can be employed to calculate bond order, including molecular orbital theory, resonance theory, and computational chemistry.
- Understanding bond order is crucial for predicting molecular properties, reactivity, and behavior in chemical reactions.
The ability to calculate bond order empowers chemists to design and synthesize new materials with tailored properties, understand reaction mechanisms, and unravel the complexities of molecular interactions in biological systems. As we continue to refine our understanding of bond order and its implications, we unlock new avenues for scientific discovery and technological advancements.
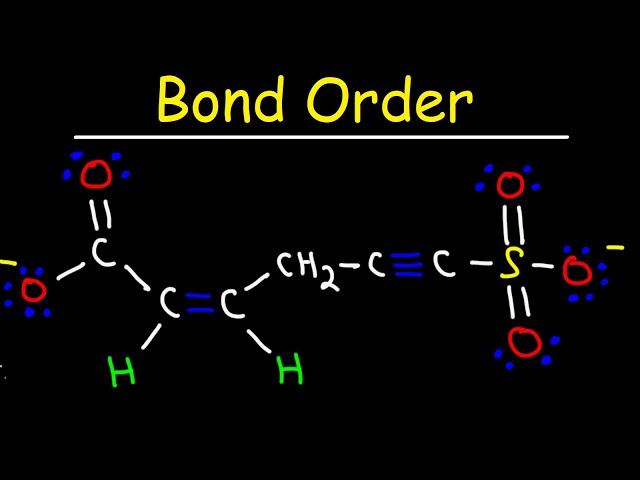