Bond order is a measure of the strength of a chemical bond between two atoms. It can be used to predict the properties of a molecule, such as its stability, reactivity, and electronic structure. Determining the bond order of diatomic molecules, which consist of two atoms, provides valuable insights into the nature of chemical bonding, molecular stability, and reactivity.
The bond order of a diatomic molecule is an important concept in chemistry that helps scientists understand the behavior and properties of molecules. It is a key factor in determining the strength and stability of a chemical bond, which in turn affects the molecule’s reactivity and other properties. The historical development of the concept of bond order dates back to the early 20th century when scientists began to explore the electronic structure of molecules.
In this article, we will explore the methods for finding the bond order of diatomic molecules, including molecular orbital theory and valence bond theory. We will also discuss the factors that affect bond order and its implications for molecular properties.
How to Find Bond Order of Diatomic Molecules
Determining the bond order of diatomic molecules is crucial for understanding their properties and behavior. Key aspects involved in finding bond order include:
- Molecular Orbital Theory
- Valence Bond Theory
- Electron Configuration
- Bond Length
- Bond Energy
- Magnetic Properties
- Reactivity
- Thermodynamic Stability
These aspects provide insights into the electronic structure, strength, and stability of the bond. Understanding bond order allows chemists to predict molecular properties, design new materials, and explore chemical reactions.
Molecular Orbital Theory
Molecular Orbital Theory is central to our understanding of chemical bonding and plays a critical role in determining the bond order of diatomic molecules. It provides a framework for describing the electronic structure of molecules and predicting their properties.
Using Molecular Orbital Theory, we can construct molecular orbitals by combining atomic orbitals. The number and type of molecular orbitals formed depend on the number and type of atomic orbitals available. The electrons in the molecule are then distributed among these molecular orbitals, following the Aufbau principle and Hund’s rule.
The bond order of a diatomic molecule is directly related to the number of electrons in the bonding molecular orbitals. A higher bond order indicates a stronger bond. Molecular Orbital Theory allows us to calculate the bond order by examining the molecular orbital diagram of the molecule. For example, in the case of the diatomic molecule hydrogen (H2), the bond order is 1, indicating a single bond between the two hydrogen atoms.
Molecular Orbital Theory is a powerful tool for understanding and predicting the properties of diatomic molecules. It provides a theoretical framework for determining bond order, which is a key factor in understanding molecular stability, reactivity, and other properties.
Valence Bond Theory
In delving into the intricacies of “how to find bond order of diatomic molecules”, Valence Bond Theory emerges as a cornerstone, providing a complementary perspective to Molecular Orbital Theory. This theory conceptualizes covalent bonding as the overlap of atomic orbitals, leading to the formation of electron pairs shared between atoms.
- Atomic Orbitals: The foundation of Valence Bond Theory lies in the hybridization of atomic orbitals, which blend to form new orbitals with specific shapes and orientations, optimizing orbital overlap and bond strength.
- Sigma and Pi Bonds: Valence Bond Theory differentiates between two types of covalent bonds: sigma () bonds, formed by head-to-head overlap of orbitals, and pi () bonds, resulting from lateral overlap. Understanding the nature of these bonds is crucial for determining bond order.
- Bond Order and Overlap: The bond order, a measure of bond strength, is directly related to the extent of orbital overlap. Greater overlap between atomic orbitals results in a higher bond order, indicating a stronger bond.
- Resonance: Valence Bond Theory allows for the description of resonance structures, which are alternative Lewis structures for a molecule that differ in the placement of double bonds and lone pairs. Resonance contributes to the overall bond order and stability of the molecule.
These facets of Valence Bond Theory provide a solid foundation for comprehending and predicting the bond order of diatomic molecules. By considering the hybridization of atomic orbitals, the formation of sigma and pi bonds, the relationship between overlap and bond order, and the concept of resonance, chemists can gain valuable insights into the electronic structure and properties of diatomic molecules.
Electron Configuration
Electron configuration, the distribution of electrons in atomic orbitals, plays a crucial role in determining the bond order of diatomic molecules. It provides insights into the number and type of bonds formed between atoms, influencing molecular properties and reactivity.
- Atomic Orbitals: Electrons occupy specific orbitals around the atomic nucleus, each with a unique shape and energy level. The arrangement of electrons in these orbitals, known as the electron configuration, determines the chemical properties of an element.
- Valence Electrons: Electrons in the outermost shell of an atom, called valence electrons, are primarily involved in chemical bonding. The number and arrangement of valence electrons determine the bonding behavior of an atom.
- Bond Formation: Atoms with unpaired valence electrons tend to form bonds to achieve a stable electron configuration, typically a filled valence shell. The overlap of atomic orbitals containing unpaired electrons leads to the formation of molecular orbitals and the sharing of electrons between atoms.
- Molecular Orbitals: The combination of atomic orbitals gives rise to molecular orbitals, which describe the distribution of electrons in a molecule. The number and type of molecular orbitals formed depend on the electron configurations of the constituent atoms.
Understanding electron configuration allows chemists to predict the bond order of diatomic molecules by examining the number and arrangement of valence electrons. This information helps determine the strength and stability of the bond, as well as the overall reactivity and properties of the molecule.
Bond Length
Bond length is a critical component of “how to find bond order of diatomic molecules” because it is directly related to the strength of the bond. Bond order is a measure of the number of electron pairs shared between two atoms, and it is inversely proportional to bond length. This means that the shorter the bond length, the higher the bond order, and the stronger the bond.
For example, the bond length of the carbon-carbon bond in ethane is 1.54 , and the bond order is 1. This means that there is one electron pair shared between the two carbon atoms, and the bond is a single bond. In contrast, the bond length of the carbon-carbon bond in ethene is 1.34 , and the bond order is 2. This means that there are two electron pairs shared between the two carbon atoms, and the bond is a double bond.
The bond length can be used to predict the bond order of a diatomic molecule. By measuring the bond length, chemists can determine the number of electron pairs shared between the two atoms and, therefore, the bond order.
Bond Energy
Bond energy plays a pivotal role in determining the bond order of diatomic molecules. It represents the strength of the chemical bond between two atoms and influences various molecular properties and chemical reactions.
- Bond Dissociation Energy: The energy required to break a bond between two atoms, providing insights into bond strength and stability.
- Bond Length: A shorter bond length typically indicates a stronger bond and higher bond energy, reflecting the inverse relationship between these parameters.
- Bond Order: Bond energy is directly proportional to bond order, meaning stronger bonds (higher bond order) have higher bond energies.
- Resonance: Resonance structures contribute to bond energy by delocalizing electrons, resulting in lower bond energy compared to localized double bonds.
Understanding bond energy allows chemists to predict the bond order of diatomic molecules and assess their stability. It also helps explain chemical reactivity, as stronger bonds are less reactive and vice versa. The interplay between bond energy and bond order provides valuable insights into molecular structure and behavior.
Magnetic Properties
Magnetic properties play a significant role in understanding the bond order of diatomic molecules. The magnetic properties of a molecule arise from the presence of unpaired electrons, which create a magnetic field. The number of unpaired electrons in a molecule is directly related to its bond order.
For example, oxygen (O2) has two unpaired electrons and is paramagnetic, meaning it is attracted to a magnetic field. This indicates that O2 has a bond order of 2, as each unpaired electron contributes to the formation of one bond. In contrast, nitrogen (N2) has no unpaired electrons and is diamagnetic, meaning it is repelled by a magnetic field. This indicates that N2 has a bond order of 3, as all of the electrons are paired.
Understanding the magnetic properties of diatomic molecules allows chemists to determine their bond order and gain insights into their electronic structure. This information is crucial for predicting molecular properties, such as reactivity and stability. Additionally, magnetic properties find applications in various fields, including magnetic resonance imaging (MRI) and nuclear magnetic resonance (NMR) spectroscopy.
Reactivity
Reactivity is a critical component of “how to find bond order of diatomic molecules” as it provides insights into the chemical behavior and stability of these molecules. Bond order, a measure of the strength of the bond between two atoms, directly influences the reactivity of a diatomic molecule.
A higher bond order generally indicates a stronger bond, which in turn leads to lower reactivity. This is because stronger bonds require more energy to break, making the molecule less likely to react with other species. For example, diatomic nitrogen (N2) has a bond order of 3 and is known for its low reactivity due to its strong triple bond. In contrast, diatomic oxygen (O2) has a bond order of 2 and is more reactive due to its weaker double bond.
Understanding the relationship between bond order and reactivity is crucial in various fields, including chemistry, materials science, and biochemistry. By determining the bond order of a diatomic molecule, scientists can predict its reactivity and design materials with desired properties. For instance, in catalysis, bond order manipulation is essential for optimizing the activity and selectivity of catalysts.
In summary, reactivity is a key aspect of “how to find bond order of diatomic molecules” because it allows chemists to understand the chemical behavior and stability of these molecules. The bond order provides valuable insights into the reactivity of diatomic molecules, enabling researchers to design materials and optimize chemical processes.
Thermodynamic Stability
Thermodynamic stability plays a crucial role in understanding the behavior of diatomic molecules and determining their bond order. It encompasses various aspects that influence the stability and properties of these molecules.
- Bond Energy: The bond energy, which represents the strength of the bond between two atoms, directly affects the thermodynamic stability of a diatomic molecule. Stronger bonds, characterized by higher bond energies, result in more stable molecules.
- Bond Length: Bond length is inversely related to bond strength and stability. Shorter bonds indicate stronger interactions between atoms, leading to increased stability. For instance, the C-C bond in ethane is shorter and more stable than the C-C bond in ethene.
- Molecular Geometry: The geometry of a diatomic molecule can impact its thermodynamic stability. Linear molecules, such as diatomic hydrogen (H2), are generally more stable than bent or nonlinear molecules due to reduced steric hindrance and optimized orbital overlap.
- Intermolecular Forces: The presence of intermolecular forces, such as van der Waals forces or hydrogen bonding, can influence the stability of diatomic molecules. These forces can stabilize molecules by promoting aggregation or association.
Understanding thermodynamic stability allows chemists to predict the properties and behavior of diatomic molecules and design materials with desired stabilities. By considering factors such as bond energy, bond length, molecular geometry, and intermolecular forces, scientists can manipulate and optimize the stability of these molecules for various applications.
Frequently Asked Questions
This FAQ section provides concise answers to commonly asked questions and clarifies key concepts related to “how to find bond order of diatomic molecules”.
Question 1: What is bond order and why is it important?
Bond order measures the strength of a bond between two atoms and provides insights into molecular properties, stability, and reactivity. A higher bond order indicates a stronger bond.
Question 2: How can I determine the bond order of a diatomic molecule using molecular orbital theory?
Molecular orbital theory involves constructing molecular orbitals by combining atomic orbitals. The number of electrons in the bonding molecular orbitals corresponds to the bond order.
Question 3: How does bond length relate to bond order?
Bond length is inversely proportional to bond order. A shorter bond length indicates a stronger bond and a higher bond order.
Question 4: Can magnetic properties provide information about bond order?
Yes, the presence of unpaired electrons in a diatomic molecule gives rise to magnetic properties. The number of unpaired electrons is directly related to the bond order.
Question 5: How does bond order influence the reactivity of a diatomic molecule?
Bond order is inversely related to reactivity. Diatomic molecules with higher bond orders are less reactive due to the stronger bonds, requiring more energy to break.
Question 6: What factors affect the bond order of a diatomic molecule?
Factors such as electron configuration, hybridization, number of valence electrons, and resonance can influence the bond order of a diatomic molecule.
These FAQs provide a concise overview of key concepts related to “how to find bond order of diatomic molecules”. Understanding bond order is crucial for comprehending molecular structure, properties, and reactivity.
In the next section, we will delve deeper into advanced methods for determining bond order and explore its applications in various fields of chemistry.
Tips for Finding Bond Order of Diatomic Molecules
This section provides practical tips to assist in accurately determining the bond order of diatomic molecules, aiding in the comprehension of molecular structure and properties.
Tip 1: Utilize Molecular Orbital Theory: Construct molecular orbitals by combining atomic orbitals and count the electrons in the bonding orbitals to determine the bond order.
Tip 2: Examine Electron Configuration: Analyze the electron configuration of the atoms involved, considering unpaired electrons and the number of valence electrons to predict bond order.
Tip 3: Measure Bond Length: Determine the bond length experimentally using techniques like X-ray crystallography or microwave spectroscopy. A shorter bond length indicates a higher bond order.
Tip 4: Consider Magnetic Properties: Observe the molecule’s magnetic behavior. Paramagnetism suggests unpaired electrons and can provide insights into bond order.
Tip 5: Determine Bond Energy: Calculate the bond energy using experimental techniques like calorimetry. Higher bond energy corresponds to a higher bond order.
Tip 6: Analyze Vibrational Frequency: Measure the vibrational frequency of the molecule using infrared or Raman spectroscopy. Higher vibrational frequencies indicate stronger bonds and higher bond orders.
Tip 7: Use Valence Bond Theory: Describe the bond formation in terms of overlapping atomic orbitals. The number and type of atomic orbitals involved can help determine bond order.
Tip 8: Consider Resonance: Examine the resonance structures of the molecule. Resonance can affect the bond order by delocalizing electrons and altering the bond strengths.
These tips provide a practical framework for determining bond order, enabling a deeper understanding of chemical bonding and molecular properties. They lay the groundwork for the concluding section, which will explore advanced applications of bond order in various fields of chemistry.
By implementing these tips, chemists can effectively find the bond order of diatomic molecules, unlocking valuable insights into molecular structure, stability, and reactivity.
Conclusion
This comprehensive guide has explored various approaches to determine the bond order of diatomic molecules, providing a deeper understanding of chemical bonding and molecular properties. Key concepts, such as electron configuration, molecular orbital theory, bond length, and magnetic properties, play crucial roles in accurately finding bond order.
The strong correlation between bond order and molecular properties, including stability, reactivity, and vibrational frequency, underscores its significance in understanding chemical behavior. By utilizing the discussed tips and methods, chemists can effectively determine bond order, enabling them to predict and manipulate molecular properties for desired outcomes.
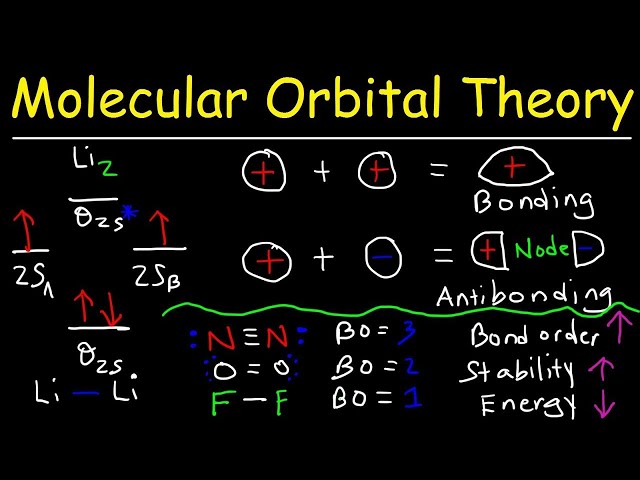