Determining the bond order is key to comprehending a molecule’s bonding and stability. It reflects the number of chemical bonds linking a pair of atoms. For instance, the double bond in molecular oxygen (O2) signifies that the oxygen atoms are connected by two shared electron pairs.
Knowledge of bond orders enables chemists to predict the reactivity, geometry, and physical properties of molecules. This concept traces its roots to the 1931 discovery of Linus Pauling, who introduced the idea of bond order as part of his groundbreaking valence bond theory.
Delving into the intricacies of bond order chemistry will empower you with the tools to understand and harness the forces that shape molecular interactions.
How to Find Bond Order Chemistry
Understanding bond order is crucial for comprehending molecular structure and reactivity. Key aspects to consider include:
- Atomic Orbitals
- Electron Configuration
- Molecular Orbital Theory
- Valence Bond Theory
- Resonance
- Hybridization
- Delocalization
- Bond Length
- Bond Energy
These aspects are interconnected and provide insights into the nature of chemical bonds. For instance, atomic orbitals describe the spatial distribution of electrons, while molecular orbital theory explains how atomic orbitals combine to form molecular orbitals. Understanding resonance and hybridization helps determine the stability and geometry of molecules. Bond length and bond energy provide information about the strength and reactivity of bonds.
Atomic Orbitals
Atomic orbitals play a central role in determining bond order and, consequently, the chemical properties of molecules. Orbitals are mathematical functions that describe the three-dimensional space around an atom where electrons are most likely to be found. Each atomic orbital corresponds to a specific energy level and shape, which influences how it interacts with other orbitals to form chemical bonds.
In the context of bond order chemistry, atomic orbitals provide the foundation for understanding the formation and properties of molecular orbitals. Molecular orbitals are formed by the overlap of atomic orbitals, and their symmetry and energy levels determine the bond order. For example, in a double bond, two atomic orbitals overlap to form a molecular orbital with a bond order of two, indicating a strong bond between the two atoms.
Understanding atomic orbitals is crucial for predicting and explaining the behavior of molecules. It allows chemists to determine the geometry, stability, and reactivity of molecules, as well as their magnetic and spectroscopic properties. This understanding has practical applications in various fields, including materials science, drug design, and catalysis.
Electron Configuration
Electron configuration plays a pivotal role in determining bond order chemistry by dictating the number and arrangement of electrons in an atom’s orbitals. The bond order, in turn, influences the strength, length, and properties of chemical bonds.
The electron configuration of an atom provides a blueprint for its chemical behavior. It determines the number of valence electrons, which are the electrons in the outermost energy level. Valence electrons participate in chemical bonding, forming bonds with other atoms to achieve a stable electron configuration.
For example, carbon has an electron configuration of 1s22s22p2. This means it has four valence electrons, two in the 2s orbital and two in the 2p orbitals. In methane (CH4), carbon forms four single bonds with hydrogen atoms. Each carbon-hydrogen bond is formed by the overlap of a carbon 2p orbital with a hydrogen 1s orbital. The resulting molecular orbitals have a bond order of one, indicating single bonds between carbon and hydrogen.
Understanding electron configuration is essential for predicting and explaining the behavior of molecules. It allows chemists to determine the types of bonds that can be formed, the geometry of molecules, and their overall stability. This understanding has practical applications in various fields, including materials science, drug design, and catalysis.
Molecular Orbital Theory
Within the realm of “how to find bond order chemistry,” Molecular Orbital Theory (MOT) stands as a cornerstone. MOT offers a quantum mechanical framework for comprehending the electronic structure of molecules, including bond formation and properties.
- Linear Combination of Atomic Orbitals (LCAO): MOT posits that molecular orbitals are constructed by combining atomic orbitals. The resulting molecular orbitals extend across the entire molecule, influencing bond order and properties.
- Symmetry and Overlap: The symmetry and overlap of atomic orbitals determine the shape and energy of molecular orbitals. Overlapping orbitals with similar symmetry can interact constructively, forming bonding molecular orbitals, while those with opposite symmetry interact destructively, forming antibonding molecular orbitals.
- Molecular Orbital Energy Levels: The energy levels of molecular orbitals are crucial for understanding chemical bonding. Bonding molecular orbitals are lower in energy than the atomic orbitals from which they are formed, while antibonding molecular orbitals are higher in energy.
- Bond Order and Molecular Properties: The bond order, a measure of bond strength, is directly related to the number of electrons occupying bonding molecular orbitals. Higher bond orders indicate stronger bonds and shorter bond lengths.
MOT provides a powerful tool for predicting and explaining various molecular properties, including bond lengths, bond strengths, and reactivity. It forms the foundation for understanding the electronic structure of molecules and is essential for fields such as quantum chemistry, materials science, and drug design.
Valence Bond Theory
Within the realm of “how to find bond order chemistry,” Valence Bond Theory (VBT) stands as a cornerstone, providing a qualitative approach to comprehending chemical bonding and molecular structure.
- Atomic Orbitals and Hybridization: VBT postulates that atomic orbitals undergo hybridization to form new orbitals with specific shapes and energies, which then participate in bond formation.
- Overlap and Resonance: VBT emphasizes the overlap between hybridized orbitals to form sigma and pi bonds. Resonance structures, involving the delocalization of electrons, are used to explain the bonding in certain molecules.
- Bond Order and Strength: VBT relates bond order to bond strength, proposing that bonds with higher bond orders are shorter and stronger. This concept helps predict the stability and reactivity of molecules.
- Magnetic Properties: VBT can be used to explain the magnetic properties of molecules. Paramagnetism arises from the presence of unpaired electrons in molecular orbitals, while diamagnetism results from all electrons being paired.
VBT offers a powerful tool for visualizing and understanding chemical bonding. Its concepts are fundamental to predicting molecular geometry, bond strengths, and magnetic properties. VBT complements Molecular Orbital Theory, providing an alternative perspective on the electronic structure of molecules.
Resonance
In the context of “how to find bond order chemistry,” resonance plays a significant role. It’s a concept that describes the delocalization of electrons across multiple equivalent Lewis structures, providing a more accurate representation of a molecule’s electronic structure.
- Equivalent Lewis Structures: Resonance involves drawing multiple Lewis structures for a molecule that differ only in the placement of electrons. These structures have the same number of bonds and atoms but vary in the distribution of double and single bonds.
- Electron Delocalization: In resonance structures, electrons are not confined to a specific bond but are spread out or “delocalized” over several atoms. This delocalization results from the overlap of atomic orbitals, allowing electrons to move freely within the molecule.
- Bond Order: Resonance can affect the bond order of a molecule. By delocalizing electrons, resonance can increase the bond order between certain atoms, making the bonds shorter and stronger.
- Stability: Resonance contributes to the stability of molecules by lowering their overall energy. Molecules with resonance structures are more stable than those without, as the delocalization of electrons reduces the repulsion between negatively charged electrons.
Understanding resonance is essential for accurately predicting the electronic structure and properties of molecules. It provides insights into bond lengths, bond strengths, and molecular stability, and is a fundamental concept in chemistry.
Hybridization
In the context of “how to find bond order chemistry,” hybridization plays a pivotal role in determining the electronic structure and properties of molecules. It involves the mixing of atomic orbitals to form new hybrid orbitals with distinct shapes and energies, which subsequently participate in chemical bonding.
- Types of Hybridization: Hybridization can occur in various forms, such as sp, sp2, sp3, and sp3d, depending on the number and type of atomic orbitals involved.
- Molecular Geometry: The type of hybridization directly influences the molecular geometry and bond angles. For instance, sp3 hybridization results in tetrahedral geometry, while sp2 hybridization leads to trigonal planar geometry.
- Bond Strength and Length: Hybridization affects the strength and length of chemical bonds. Hybrid orbitals with greater overlap have stronger and shorter bonds.
- Reactivity: Hybridization can influence the reactivity of molecules. Hybrid orbitals with higher energy are more reactive and participate more readily in chemical reactions.
Understanding hybridization is crucial for accurately predicting the structure, bonding, and properties of molecules. It provides insights into a wide range of chemical phenomena, from molecular geometry to reactivity, and is a fundamental concept in “how to find bond order chemistry.”
Delocalization
Delocalization is a crucial concept in “how to find bond order chemistry” as it describes the spreading out or delocalization of electrons over multiple atoms within a molecule or ion. This phenomenon plays a significant role in determining the electronic structure, bonding, and properties of molecules.
- Resonance: Delocalization often occurs through resonance, where multiple Lewis structures can be drawn for a molecule, each with a different arrangement of double and single bonds. This delocalization of electrons contributes to the stability of molecules.
- Pi-Bonding: Delocalization is common in molecules with pi-bonds, where electrons are shared between atoms in a p-orbital overlap fashion. The delocalized electrons create a continuous electron cloud above and below the plane of the atoms.
- Aromatic Compounds: Delocalization is a defining characteristic of aromatic compounds, such as benzene. The delocalized electrons in the pi-system contribute to the stability and unique properties of aromatic compounds.
- Metallic Bonding: In metals, delocalization occurs through the sharing of valence electrons among all the atoms in the metallic lattice. This delocalization results in high electrical and thermal conductivity.
Understanding delocalization is essential for accurately predicting the electronic structure and properties of molecules. It provides insights into molecular stability, bonding, reactivity, and various physical and chemical phenomena.
Bond Length
Delving into “how to find bond order chemistry” necessitates an examination of “Bond Length,” a crucial factor influencing bond strength, molecular geometry, and overall molecular properties.
- Interatomic Distance: Bond length refers to the distance between the nuclei of two bonded atoms. It is a direct measure of the strength of the bond, with shorter bonds indicating stronger interactions.
- Bond Order: Bond length is inversely proportional to bond order. Higher bond orders correspond to shorter bond lengths due to increased electron density between the bonded atoms.
- Hybridization: The type of hybridization of atomic orbitals affects bond length. For instance, sp3 hybridized orbitals result in shorter and stronger bonds compared to sp2 hybridized orbitals.
- Electronegativity: The electronegativity of the bonded atoms influences bond length. Bonds between atoms with a significant electronegativity difference tend to be longer and weaker.
Understanding bond length provides valuable insights into the nature of chemical bonds and their impact on molecular behavior. By considering bond length in conjunction with other factors such as bond order and hybridization, chemists can accurately predict molecular structures and properties, guiding the design and synthesis of new materials with tailored functionalities.
Bond Energy
In the realm of “how to find bond order chemistry,” “Bond Energy” stands as a cornerstone, providing critical insights into the strength and stability of chemical bonds. It quantifies the energy required to break a bond between two atoms and offers valuable information about molecular structure, reactivity, and various chemical processes.
- Bond Strength: Bond energy directly relates to bond strength; stronger bonds have higher bond energies, indicating greater resistance to bond breaking.
- Bond Type: Different types of bonds, such as single, double, and triple bonds, exhibit varying bond energies. Multiple bonds possess higher bond energies due to the increased number of shared electron pairs.
- Electronegativity: The electronegativity of bonded atoms influences bond energy. Bonds between atoms with significant electronegativity differences tend to have lower bond energies due to the polarization of the electron cloud.
- Bond Length: Bond energy is inversely proportional to bond length. Shorter bonds typically have higher bond energies as the atoms are held more closely together.
Understanding bond energy is paramount in predicting the stability and reactivity of molecules. By considering bond energies in conjunction with other factors such as bond order and hybridization, chemists can gain a comprehensive understanding of chemical bonding and its implications in various chemical processes.
Frequently Asked Questions (FAQs)
This section addresses commonly asked questions and clarifies key aspects of “how to find bond order chemistry.”
Question 1: What is bond order?
Bond order refers to the number of chemical bonds between two atoms, providing insights into bond strength and molecular stability.
Question 2: How is bond order related to bond length?
Bond order is inversely related to bond length; higher bond orders correspond to shorter bond lengths due to increased electron density.
Question 3: What factors influence bond order?
Bond order is influenced by various factors, including atomic orbital overlap, hybridization, and the number of shared electrons.
Question 4: How can I determine the bond order of a molecule?
To find the bond order, one can use various methods such as Lewis dot structures, molecular orbital theory, and valence bond theory.
Question 5: What is the significance of bond order in chemistry?
Bond order plays a crucial role in understanding molecular properties, predicting reactivity, and designing new materials with desired functionalities.
Question 6: How is bond order related to resonance and delocalization?
Resonance and delocalization can affect bond order by distributing electrons over multiple atoms, resulting in fractional bond orders and increased molecular stability.
These FAQs provide a concise overview of key concepts related to “how to find bond order chemistry.” Understanding these concepts is essential for further exploration of chemical bonding and its applications.
In the next section, we will delve deeper into the applications of bond order in predicting molecular properties and chemical reactivity.
Tips for Understanding Bond Order Chemistry
To enhance your comprehension of bond order chemistry, consider implementing these practical tips:
Tip 1: Start with a Strong Foundation: Begin by solidifying your understanding of atomic orbitals, electron configurations, and molecular orbital theory.
Tip 2: Practice Drawing Lewis Structures: Visualizing molecular structures through Lewis dot diagrams helps determine bond order and identify resonance.
Tip 3: Employ Valence Bond Theory: Utilize VBT to analyze bond formation and hybridization, providing insights into bond strength and geometry.
Tip 4: Consider Molecular Orbital Interactions: Understand how atomic orbitals combine to form molecular orbitals, influencing bond order and molecular properties.
Tip 5: Delve into Delocalization: Recognize the importance of delocalization in resonance structures, affecting bond order and molecular stability.
Tip 6: Examine Bond Lengths and Energies: Analyze the relationships between bond order, bond length, and bond energy to predict molecular properties.
By incorporating these tips into your study approach, you can effectively grasp the intricacies of bond order chemistry and its applications.
In the following section, we will explore advanced concepts related to bond order, providing a comprehensive understanding of this fundamental aspect of chemistry.
Conclusion
This comprehensive exploration of “how to find bond order chemistry” elucidated the critical aspects of bond formation and molecular properties. Understanding bond order provides valuable insights into chemical bonding, molecular geometry, and reactivity. Key concepts explored include atomic orbital interactions, hybridization, resonance, and their impact on bond length and energy.
The interconnections between these concepts highlight the complexity and elegance of chemical bonding. Bond order analysis empowers chemists to predict molecular behavior, design new materials, and unravel the mechanisms of chemical reactions. As we continue to delve into the realm of chemistry, the concept of bond order remains a cornerstone for comprehending the fundamental forces that govern the molecular world.
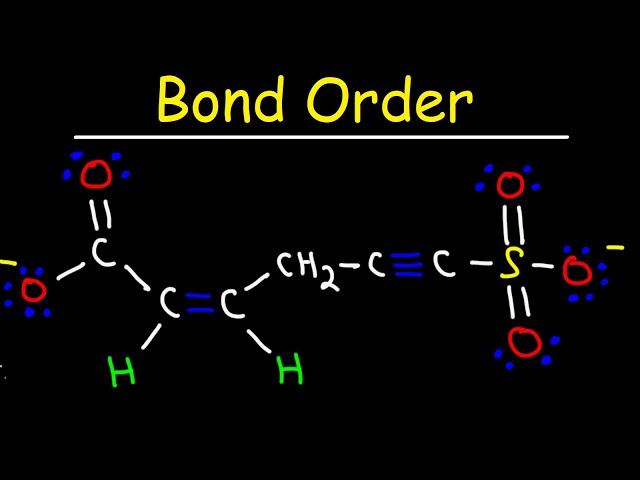