Determining Bond Order: Unveiling Chemical Bond Strength
In chemistry, bond order plays a pivotal role in understanding the nature and strength of chemical bonds. It provides valuable insights into the stability, reactivity, and properties of molecules. Historically, the concept of bond order evolved from the seminal work of Linus Pauling, who proposed that the strength of a chemical bond is directly related to its order.
Calculating bond order involves examining the electron configuration of molecules and the number of shared electron pairs between atoms. It allows chemists to predict the bond length, bond energy, and magnetic properties of various compounds. This knowledge is crucial in fields such as molecular spectroscopy, catalysis, and pharmaceutical development.
Calculating Bond Order in Chemistry
Understanding bond order is crucial for deciphering chemical bond strength and molecular properties. Key aspects involved in calculating bond order chemistry encompass:
- Electron Configuration
- Molecular Orbitals
- Shared Electron Pairs
- Bond Length
- Bond Energy
- Magnetic Properties
- Resonance Structures
- Hybridization
These aspects provide a comprehensive framework for comprehending bond order’s influence on molecular stability, reactivity, and various chemical phenomena.
Electron Configuration
Electron configuration plays a crucial role in calculating bond order chemistry. It refers to the distribution of electrons in atomic orbitals, which determines the chemical properties and bonding behavior of an atom. For instance, carbon’s electron configuration (1s22s22p2) indicates that it has two electrons in the valence p-orbitals, enabling it to form four covalent bonds with other atoms.
In the context of bond order calculation, electron configuration dictates the number of unpaired electrons available for bonding. For example, in the formation of a double bond between two carbon atoms, each carbon contributes one unpaired electron from its 2p-orbitals. These unpaired electrons combine to form a shared electron pair, resulting in a bond order of two.
Understanding electron configuration is pivotal for calculating bond order accurately. It provides insights into the stability, reactivity, and magnetic properties of various compounds. This knowledge finds applications in diverse fields such as materials science, catalysis, and drug design.
Molecular Orbitals
Molecular orbitals are mathematical functions that describe the wave-like behavior of electrons in molecules. They are crucial for understanding and calculating bond order chemistry because they dictate the number and type of chemical bonds that can form between atoms. Molecular orbitals arise from the combination of atomic orbitals, which are the orbitals of individual atoms.
When atoms bond, their atomic orbitals overlap to form molecular orbitals. The number of molecular orbitals formed is equal to the total number of atomic orbitals that overlap. The shapes and energies of the molecular orbitals depend on the symmetry and overlap of the atomic orbitals. The electrons in the molecule occupy the molecular orbitals in order of increasing energy.
The molecular orbitals involved in bond formation are called bonding orbitals. Bonding orbitals have lower energy than the atomic orbitals from which they are formed. This energy difference is the bond energy, which is the energy required to break the bond. The bond order is equal to the number of electrons in bonding orbitals minus the number of electrons in antibonding orbitals. Antibonding orbitals are molecular orbitals that have higher energy than the atomic orbitals from which they are formed.
Understanding molecular orbitals is critical for calculating bond order chemistry because it allows us to predict the strength and type of chemical bonds that can form between atoms. This knowledge is essential for understanding the structure and properties of molecules.
Shared Electron Pairs
Shared electron pairs play a pivotal role in calculating bond order chemistry. Bond order, a measure of the strength of a chemical bond, is directly related to the number of shared electron pairs between atoms. A shared electron pair is a pair of electrons that occupies a molecular orbital that is formed by the overlap of atomic orbitals from two different atoms. The more shared electron pairs there are between two atoms, the stronger the bond between them.
To calculate bond order, we need to determine the number of shared electron pairs between the two atoms in question. This can be done by examining the molecular orbital diagram for the molecule. The molecular orbital diagram shows the energy levels of the molecular orbitals and the number of electrons that occupy each orbital. The number of shared electron pairs is equal to the number of electrons that occupy the bonding molecular orbitals.
Shared electron pairs are essential for understanding and predicting the properties of molecules. The number of shared electron pairs between two atoms determines the bond order, which in turn affects the bond length, bond strength, and other properties of the molecule. For example, a double bond, which is formed by sharing two pairs of electrons, is stronger and shorter than a single bond, which is formed by sharing only one pair of electrons.
Understanding shared electron pairs is crucial for a variety of applications in chemistry, including predicting the reactivity of molecules, designing new materials, and understanding the behavior of biological systems. By understanding the relationship between shared electron pairs and bond order, chemists can gain valuable insights into the structure and properties of matter.
Bond Length
Bond length, an essential aspect of bond order chemistry, refers to the distance between the nuclei of two bonded atoms. It is inversely related to bond order, meaning that the higher the bond order, the shorter the bond length. Understanding bond length is crucial for predicting the properties and reactivity of molecules.
- Atomic Radii: The radii of the bonded atoms influence the bond length. Larger atoms generally form longer bonds than smaller atoms.
- Bond Order: As mentioned earlier, bond order and bond length are inversely proportional. Double bonds are shorter than single bonds, and triple bonds are shorter than double bonds.
- Hybridization: The hybridization of the atomic orbitals involved in bonding affects the bond length. For instance, sp hybridized orbitals lead to shorter bond lengths than sp3 hybridized orbitals.
- Electronegativity: The difference in electronegativity between the bonded atoms can affect the bond length. Bonds between atoms with a large electronegativity difference are generally shorter than bonds between atoms with a small electronegativity difference.
Understanding bond length is essential for predicting the properties and reactivity of molecules. It provides insights into the strength, polarity, and vibrational frequencies of chemical bonds. By considering the factors that influence bond length, chemists can gain a deeper understanding of molecular structure and behavior.
Bond Energy
Delving into bond order chemistry, bond energy stands as a crucial aspect, influencing molecular stability and chemical reactions. It represents the energy required to break a bond between two atoms, providing insights into the strength and reactivity of chemical bonds.
- Bond Strength: Bond energy directly correlates with bond strength – higher bond energy indicates a stronger bond. This strength influences various molecular properties, such as melting and boiling points.
- Bond Type: Different types of bonds, such as single, double, or triple bonds, exhibit varying bond energies. Understanding these energies aids in predicting the reactivity and stability of molecules.
- Chemical Reactions: Bond energy plays a critical role in determining the feasibility and outcome of chemical reactions. Reactions involving bonds with lower bond energies are generally more likely to occur.
- Molecular Structure: Bond energies influence molecular geometry and shape. Molecules adopt configurations that minimize bond energy, leading to stable and predictable structures.
Comprehending bond energy is pivotal in understanding and predicting chemical behavior. By examining its various facets and implications, chemists gain valuable insights into the nature and dynamics of chemical bonds, enabling them to design and manipulate molecules for specific applications.
Magnetic Properties
Within the realm of bond order chemistry, magnetic properties offer valuable insights into the nature of chemical bonds. These properties arise from the magnetic moments of electrons and provide information about the electronic structure and bonding of molecules.
- Paramagnetism:
Paramagnetic substances have unpaired electrons, resulting in a net magnetic moment. This property is observed in molecules with odd numbers of electrons or transition metal complexes.
- Diamagnetism:
Diamagnetic substances have all electrons paired, leading to a zero net magnetic moment. This property is common in molecules with closed-shell electron configurations.
- Ferromagnetism:
Ferromagnetic substances exhibit a strong magnetic attraction due to the alignment of unpaired electrons in neighboring atoms. This property is found in materials like iron, nickel, and cobalt.
- Antiferromagnetism:
Antiferromagnetic substances have unpaired electrons aligned in an antiparallel fashion, resulting in a cancellation of the magnetic moments. This property is observed in certain metal oxides and minerals.
Understanding magnetic properties is crucial for elucidating the electronic structure of molecules and materials. By analyzing the magnetic behavior of substances, chemists can gain insights into bond order, molecular symmetry, and the presence of unpaired electrons. This knowledge finds applications in various fields, including materials science, catalysis, and the development of magnetic resonance imaging techniques.
Resonance Structures
Resonance structures are a fundamental concept in bond order chemistry, providing a means to describe the delocalization of electrons within a molecule or ion. By considering multiple contributing structures, resonance structures offer a more accurate representation of the bonding and molecular properties.
- Equivalent Contributors: Resonance structures are not individual structures but rather different representations of the same molecule or ion. Each structure contributes to the overall resonance hybrid, which is a weighted average of the individual contributors.
- Electron Delocalization: Resonance structures depict the delocalization of electrons, where electrons are not confined to a specific atom or bond but are spread over several atoms. This delocalization results in a more stable and lower-energy molecular system.
- Bond Order: Resonance structures can affect bond order calculations. By considering the different resonance contributors, the calculated bond order may be an average or fractional value, reflecting the delocalization of electrons.
- Examples: Benzene, ozone, and the carbonate ion are notable examples of molecules and ions where resonance structures play a crucial role in understanding their bonding and properties.
Resonance structures provide a deeper understanding of molecular bonding and properties. By considering the delocalization of electrons and the contributions of multiple resonance structures, chemists can gain insights into molecular stability, reactivity, and various chemical phenomena.
Hybridization
Hybridization plays a pivotal role in understanding and calculating bond order chemistry. It refers to the process of combining atomic orbitals to form new hybrid orbitals with different shapes and energies. Hybridization influences the geometry, bonding, and properties of molecules.
In the context of bond order calculations, hybridization determines the number and type of atomic orbitals that overlap to form molecular orbitals. The type of hybridization also affects the strength and polarity of the resulting bonds. For instance, sp3 hybridization leads to tetrahedral geometry and stronger sigma bonds, while sp2 hybridization results in trigonal planar geometry and weaker pi bonds.
Real-life examples of hybridization in bond order chemistry include the double bond in ethene (C2H4) and the triple bond in acetylene (C2H2). In ethene, the carbon atoms undergo sp2 hybridization, forming three sigma bonds (two C-H bonds and one C-C bond) and one pi bond. In acetylene, the carbon atoms undergo sp hybridization, forming two sigma bonds (two C-H bonds) and two pi bonds.
Understanding hybridization is crucial for accurately calculating bond order and predicting molecular properties. It provides insights into the stability, reactivity, and spectroscopic behavior of molecules. This understanding finds applications in various fields, including organic chemistry, inorganic chemistry, and materials science.
Frequently Asked Questions about Bond Order Chemistry
This section addresses common questions and clarifies concepts related to calculating bond order chemistry.
Question 1: What is bond order, and why is it important?
Answer: Bond order represents the strength of a chemical bond and is crucial for understanding molecular stability, reactivity, and various chemical properties.
Question 2: How do I calculate bond order using the molecular orbital theory?
Answer: Bond order can be calculated as half the difference between the number of electrons in bonding and antibonding molecular orbitals.
Question 3: What factors influence bond order?
Answer: Factors such as the number of shared electrons, atomic orbital overlap, and hybridization affect bond order.
Question 4: How is bond order related to bond length and bond energy?
Answer: Bond order is inversely proportional to bond length and directly proportional to bond energy.
Question 5: Can bond order be fractional?
Answer: Yes, bond order can be fractional in cases involving resonance or delocalization of electrons.
Question 6: What are some applications of bond order calculations?
Answer: Bond order calculations find applications in predicting molecular properties, designing new materials, and understanding chemical reactions.
These FAQs provide essential insights into the concepts and applications of bond order chemistry. Understanding bond order enables chemists to delve deeper into the nature of chemical bonding and its implications in various fields of science.
In the next section, we will explore advanced topics in bond order chemistry, including resonance and hybridization effects.
Tips for Mastering Bond Order Chemistry
This section presents practical tips to enhance your understanding and problem-solving skills in bond order chemistry.
Tip 1: Utilize molecular orbital theory to visualize the formation and properties of chemical bonds.Tip 2: Analyze the symmetry and overlap of atomic orbitals to determine the type and strength of molecular orbitals formed.Tip 3: Consider the hybridization of atomic orbitals and its influence on bond angles and molecular geometry.Tip 4: Apply resonance theory to depict the delocalization of electrons and calculate resonance structures.Tip 5: Understand the relationship between bond order, bond length, and bond energy to predict molecular stability and reactivity.Tip 6: Utilize experimental techniques, such as spectroscopy and diffraction, to determine bond orders and molecular structures.
By incorporating these tips into your study, you will gain a comprehensive understanding of bond order chemistry, enabling you to analyze and predict the properties and behavior of molecules.
In the concluding section, we will explore advanced applications of bond order chemistry in various fields of science and technology.
Conclusion
This article has provided a comprehensive overview of the fundamental concepts and applications of bond order chemistry. We have explored various aspects, including electron configuration, molecular orbitals, shared electron pairs, bond length, bond energy, magnetic properties, resonance structures, and hybridization. A thorough understanding of these concepts is essential for accurately calculating bond order and predicting the properties and behavior of molecules.
Two main points stand out from our exploration:
- Bond order is a crucial parameter that governs molecular stability, reactivity, and various chemical phenomena.
- Understanding bond order requires a multifaceted approach, considering factors such as atomic orbital interactions, electron delocalization, and resonance.
These points are interconnected, as they collectively provide a comprehensive framework for analyzing and predicting chemical bonding.As we continue to delve into the realm of chemistry, it is important to remember that bond order chemistry is a fundamental pillar upon which our understanding of molecular structure and properties rests. By mastering these concepts, we empower ourselves to explore new frontiers in chemistry and related fields, paving the way for advancements in materials science, pharmaceuticals, and more.
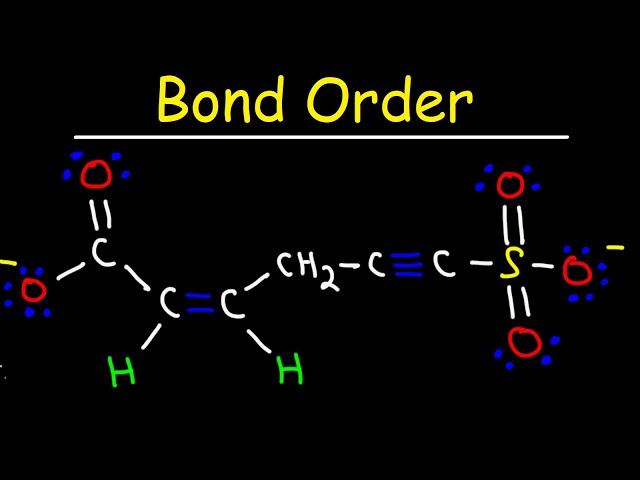