Calculating bond order, a measure of bond strength, is essential for understanding chemical bonding. Imagine a molecule like carbon dioxide (CO2). By applying the bond order formula, we can determine the strength of the double bond between carbon and each oxygen atom, offering insights into the molecule’s stability and reactivity.
Bond order calculations have profound implications in various scientific disciplines, including materials science, drug discovery, and environmental chemistry. Historically, Linus Pauling’s groundbreaking work in the 1930s laid the foundation for our understanding of bond order. His concept of “resonance” revolutionized the field, allowing us to describe the delocalization of electrons and their impact on bond strength.
In this comprehensive guide, we will delve into the intricacies of bond order formula, exploring its theoretical underpinnings, practical applications, and advanced techniques for calculating bond orders in complex molecules.
How to Calculate Bond Order Formula
Bond order formula plays a crucial role in determining the strength and stability of chemical bonds. Understanding its key aspects is essential for accurate calculations:
- Electron Configuration
- Molecular Orbitals
- Bond Length
- Bond Energy
- Resonance
- Hybridization
- Electronegativity
- Atomic Orbitals
- Quantum Mechanics
These aspects are interconnected. Electron configuration determines the number and type of atomic orbitals available for bonding, which in turn influences the formation of molecular orbitals and bond strength. Bond length and energy are directly related to bond order, while resonance and hybridization can affect the distribution of electrons and hence the bond order. Electronegativity and atomic orbitals provide insights into the polarity and overlap of electron clouds, influencing bond order calculations. Quantum mechanics underpins the theoretical framework for understanding and calculating bond order.
Electron Configuration
Electron configuration plays a central role in calculating bond order formula. It refers to the distribution of electrons in atomic orbitals, which determines the chemical properties and bonding behavior of atoms. Understanding electron configuration is essential for accurately predicting bond order and the overall stability of molecules.
- Atomic Orbitals: Electrons occupy specific orbitals around the, which have distinct shapes and energies. The type and number of atomic orbitals available depend on the element and its electron configuration.
- aufbau Principle: Electrons fill atomic orbitals in the order of increasing energy, following the aufbau principle. This principle determines the electronic configuration of atoms in their ground state.
- Hund’s Rule: When multiple atomic orbitals have the same energy, electrons occupy them singly before pairing up. This rule affects the electron configuration and influences the magnetic properties of atoms.
- Noble Gas Configuration: Atoms with a stable electron configuration, known as noble gas configuration, have their outermost energy level completely filled. This configuration results in low chemical reactivity.
Electron configuration directly influences bond formation and strength. Atoms with similar electron configurations tend to form stronger bonds, as they have a greater overlap of atomic orbitals. Conversely, atoms with significant differences in electron configuration may form weaker bonds or even repel each other. By considering the electron configurations of the atoms involved, chemists can predict the type and strength of bonds that will form.
Molecular Orbitals
Molecular orbitals play a critical role in calculating bond order formula. They are mathematical functions that describe the wave-like behavior of electrons in molecules. By combining atomic orbitals, molecular orbitals form an extended region where electrons can be found. The number and type of molecular orbitals formed depend on the number and type of atomic orbitals available.
The overlap of atomic orbitals determines the strength and type of molecular orbitals formed. Overlapping atomic orbitals with similar energies and symmetry can result in strong bonding molecular orbitals, while orbitals with different energies or symmetry may form weaker antibonding molecular orbitals. The molecular orbitals are then filled with electrons according to the Aufbau principle and Hund’s rule, similar to atomic orbitals.
The bond order is directly related to the number of bonding and antibonding electrons in a molecular orbital. A bond order of 1 indicates a single bond, 2 indicates a double bond, and so on. By calculating the bond order, chemists can predict the strength and stability of chemical bonds. This understanding is crucial in various fields, including materials science, drug design, and catalysis.
Bond Length
Bond length is a critical component of “how to calculate bond order formula” because it reflects the strength and nature of the bond between two atoms. Bond order, in turn, is a measure of the number of electron pairs shared between atoms, which influences the bond’s strength and stability. Therefore, understanding the relationship between bond length and bond order is essential for accurately calculating bond order using the appropriate formula.
In general, shorter bond lengths indicate stronger bonds, while longer bond lengths indicate weaker bonds. This relationship arises from the quantum mechanical properties of electrons, which occupy specific orbitals around atoms. When atoms form bonds, their atomic orbitals overlap, creating molecular orbitals that hold the electrons. The extent of orbital overlap determines the bond length and strength.
For example, in a double bond between two carbon atoms, the p-orbitals of each carbon overlap more significantly than in a single bond. This greater overlap leads to a shorter bond length and a stronger bond. Conversely, in a single bond, the p-orbitals overlap less, resulting in a longer bond length and a weaker bond.
Understanding the relationship between bond length and bond order has important practical applications in chemistry. For example, in materials science, it helps researchers design new materials with specific properties by controlling the bond lengths and strengths within the material. In drug design, it aids in predicting the binding affinity of drugs to target proteins by considering the bond lengths and strengths between the drug molecule and the protein.
Bond Energy
Bond energy plays a crucial role in determining the strength and stability of chemical bonds, providing valuable insights for calculating bond order accurately. It refers to the amount of energy required to break a bond between two atoms, and its magnitude is directly related to the bond order.
- Bond Strength: Bond energy is a measure of bond strength, with stronger bonds requiring more energy to break. A higher bond energy indicates a more stable bond, which influences the overall stability and reactivity of molecules.
- Bond Type: Bond energy varies depending on the type of bond formed. For example, covalent bonds, where electrons are shared between atoms, typically have lower bond energies compared to ionic bonds, where electrons are transferred from one atom to another.
- Bond Length: Bond energy is inversely related to bond length. Shorter bonds, with a greater overlap of atomic orbitals, generally have higher bond energies, indicating a stronger bond.
- Resonance: Resonance, the delocalization of electrons across multiple bonds, can affect bond energy. Delocalized electrons strengthen the overall bonding system, resulting in lower bond energies for each individual bond.
Understanding bond energy is essential for accurately calculating bond order using appropriate formulas. By considering the strength, type, length, and resonance of bonds, chemists can determine the bond order, which provides valuable insights into the electronic structure and stability of molecules.
Resonance
Resonance, a fundamental concept in chemistry, has a profound impact on “how to calculate bond order formula.” It arises from the delocalization of electrons across multiple bonds or atoms, creating hybrid structures that contribute to the overall electronic structure of a molecule. This phenomenon significantly influences bond order calculations and provides valuable insights into molecular bonding.
Resonance affects bond order formula by altering the distribution of electrons within a molecule. Delocalized electrons, spread over several atoms or bonds, strengthen the overall bonding system. As a result, each individual bond in a resonance structure has a lower bond order compared to a localized bond. To accurately calculate bond order in such cases, resonance structures must be considered to account for the delocalization of electrons.
Real-life examples of resonance abound. Benzene, a ubiquitous aromatic hydrocarbon, is a classic case. Its Kekule structure depicts alternating single and double bonds, but resonance theory suggests that the electrons are delocalized, forming a ring of continuously overlapping p-orbitals. This delocalization results in a bond order of 1.5 for each carbon-carbon bond, reflecting the resonance hybrid’s increased stability.
Understanding resonance is crucial for various practical applications. In drug design, it helps predict the reactivity and binding affinity of drugs by considering resonance effects on bond lengths, angles, and overall molecular geometry. In materials science, it aids in designing materials with specific electronic and optical properties by controlling resonance effects within the material’s structure.
Hybridization
In the context of “how to calculate bond order formula”, hybridization plays a crucial role in understanding the electronic structure and bonding behavior of molecules. Hybridization involves the intermixing of atomic orbitals to form new hybrid orbitals with unique shapes and properties, influencing the bond order and overall molecular geometry.
- Types of Hybridization
Different types of hybridization arise from the combination of atomic orbitals. Common types include sp, sp2, and sp3 hybridization, each leading to specific molecular geometries and bond angles.
- Atomic Orbital Contribution
The type of hybridization depends on the number and type of atomic orbitals involved in the hybridization process. For instance, sp3 hybridization involves the mixing of one s orbital and three p orbitals.
- Molecular Shape
Hybridization influences the molecular shape by determining the arrangement of the hybrid orbitals around the central atom. sp3 hybridization results in a tetrahedral shape, while sp2 hybridization leads to a trigonal planar shape.
- Bond Order
The overlap of hybrid orbitals affects the bond order and strength. Overlapping hybrid orbitals with greater overlap have a higher bond order, resulting in stronger bonds.
Understanding hybridization is essential for accurately calculating bond order formula. By considering the type of hybridization, the number of hybrid orbitals formed, and their overlap, chemists can determine the bond order and predict the molecular structure and bonding behavior of various molecules.
Electronegativity
Electronegativity, a crucial concept in chemistry, plays a significant role in “how to calculate bond order formula.” It refers to the ability of an atom to attract electrons towards itself within a chemical bond. Understanding electronegativity is essential for accurately calculating bond order and predicting the polarity and reactivity of molecules.
Electronegativity affects bond order by influencing the distribution of electrons within a bond. Atoms with higher electronegativity attract electrons more strongly, resulting in a shift of electron density towards them. This shift creates a polar bond, where one atom has a partial positive charge and the other has a partial negative charge. The polarity of the bond affects its strength and reactivity.
Real-life examples of electronegativity’s impact on bond order can be seen in various molecules. For instance, in hydrogen chloride (HCl), chlorine is more electronegative than hydrogen. As a result, the electron density is shifted towards chlorine, creating a polar bond with a partial positive charge on hydrogen and a partial negative charge on chlorine. This polarity influences the bond strength and reactivity of HCl.
Understanding electronegativity has practical applications in various fields. In drug design, it helps predict the binding affinity and selectivity of drugs by considering the electronegativity of the atoms involved in drug-target interactions. In materials science, it aids in designing materials with specific electrical and optical properties by controlling the electronegativity of the constituent atoms.
Atomic Orbitals
In exploring the intricate world of “how to calculate bond order formula”, “atomic orbitals” emerge as foundational building blocks, shaping the very nature of chemical bonding. Atomic orbitals, the mathematical descriptions of electron wave functions around an atom’s nucleus, play a pivotal role in determining the strength and characteristics of chemical bonds.
The types of atomic orbitals involved in bonding, such as s, p, and d orbitals, dictate the number and orientation of electron pairs that can be shared between atoms. The overlap of these atomic orbitals during bond formation creates molecular orbitals, which house the shared electrons and determine the bond’s strength and order.
Take the example of the hydrogen molecule (H2). Each hydrogen atom contributes one electron to the bond, occupying a 1s atomic orbital. As these atomic orbitals overlap head-to-head, they form a molecular orbital that accommodates both electrons. The extent of overlap and the symmetry of the molecular orbital determine the bond order, which in this case is 1, indicating a single bond.
Understanding the relationship between atomic orbitals and bond order formula has profound implications in chemistry. It enables chemists to predict molecular structures, explain chemical reactivity, and design new materials with tailored properties. This knowledge finds practical applications in diverse fields, including drug development, catalysis, and materials science, where controlling bond order is crucial for achieving desired outcomes.
Quantum Mechanics
In the realm of “how to calculate bond order formula”, “Quantum Mechanics” emerges as a fundamental pillar, providing the theoretical underpinnings that govern the behavior of electrons and their interactions within chemical bonds. This intricate framework unveils the wave-particle duality of electrons and the probabilistic nature of their behavior, offering a deeper understanding of bond formation and properties.
- Wave Functions
Quantum Mechanics describes electrons not as fixed particles but rather as waves, characterized by wave functions that determine their spatial distribution and behavior. These wave functions play a crucial role in calculating bond order, as they quantify the probability of finding electrons in specific regions around atoms.
- Atomic Orbitals
Electrons occupy specific atomic orbitals, which are defined by their shape, energy, and orientation. The overlap of these atomic orbitals during chemical bonding leads to the formation of molecular orbitals, which dictate the bond order and strength.
- Hybridization
Quantum Mechanics explains the concept of hybridization, where atomic orbitals combine to form new hybrid orbitals with distinct shapes and properties. Hybridization influences the geometry of molecules and the strength of their bonds.
- Resonance
Quantum Mechanics provides the theoretical basis for resonance, a phenomenon where electrons are delocalized across multiple bonds or atoms. This delocalization affects the bond order and stability of molecules, influencing their chemical reactivity.
By unraveling the quantum mechanical principles that govern bond formation, chemists gain invaluable insights into the electronic structure and behavior of molecules. This knowledge empowers them to accurately calculate bond order, predict molecular properties, and design materials with tailored characteristics. Quantum Mechanics remains an indispensable tool in the chemist’s arsenal, enabling a deeper understanding of the fundamental forces that shape the molecular world.
FAQs on How to Calculate Bond Order Formula
This section provides answers to frequently asked questions and clarifies common misconceptions regarding bond order formula calculations.
Question 1: What is the significance of bond order in chemistry?
Answer: Bond order is a crucial parameter that reflects the strength and stability of chemical bonds. It helps predict molecular properties, such as bond length, bond energy, and reactivity.
Question 2: How do I determine the bond order of a molecule?
Answer: Bond order can be calculated using various methods, including the Lewis dot structure method, molecular orbital theory, and valence bond theory. The specific method depends on the complexity of the molecule.
Question 3: What factors affect bond order?
Answer: Bond order is influenced by several factors, including the number of shared electrons, the electronegativity of the bonded atoms, and the hybridization of atomic orbitals.
Question 4: How does resonance affect bond order?
Answer: Resonance occurs when multiple Lewis dot structures can be drawn for a molecule. It leads to the delocalization of electrons, which can alter the bond order compared to a single Lewis structure.
Question 5: What is the relationship between bond order and bond length?
Answer: Generally, bond order is inversely proportional to bond length. Shorter bonds have higher bond orders, indicating stronger bonds.
Question 6: How can I use bond order to understand chemical reactions?
Answer: Bond order provides insights into the reactivity of molecules. Higher bond orders indicate less reactive bonds, while lower bond orders suggest more reactive bonds.
These FAQs provide a concise overview of the key aspects of bond order formula calculations. Understanding these concepts is essential for accurately predicting the properties and behavior of chemical compounds.
In the next section, we will delve deeper into advanced techniques for calculating bond order in complex molecules, exploring the intricacies of molecular orbital theory and resonance.
Tips for Calculating Bond Order Formula
To enhance your understanding and accuracy in calculating bond order formula, consider the following practical tips:
Tip 1: Determine the molecular structure and identify the atoms involved in bonding.
Tip 2: Count the number of shared electrons between the bonded atoms.
Tip 3: Consider the electronegativity of the bonded atoms to determine the polarity of the bond.
Tip 4: Utilize resonance theory to account for delocalized electrons, which can affect bond order.
Tip 5: Apply molecular orbital theory to complex molecules, where hybridization and resonance play significant roles.
Tip 6: Understand the relationship between bond order, bond length, and bond energy to gain insights into molecular stability.
Tip 7: Use computational chemistry software to assist in complex bond order calculations.
Tip 8: Continuously practice and refine your skills to master bond order formula calculations.
By following these tips, you can effectively calculate bond order, unravel the electronic structure of molecules, and gain a deeper understanding of chemical bonding.
These insights will serve as a stepping stone towards exploring advanced concepts in quantum chemistry, such as molecular orbital theory and resonance, which will be discussed in the concluding section of this article.
Conclusion
Our exploration of “how to calculate bond order formula” has unveiled the intricacies of chemical bonding, providing a deeper understanding of molecular structure and stability. Key insights include the significance of electron configuration, molecular orbitals, and resonance in determining bond order. These concepts are interconnected, with electron configuration influencing the formation of molecular orbitals, which in turn affect bond strength and resonance.
Understanding bond order formula empowers chemists to predict molecular properties, design new materials, and unravel the mechanisms of chemical reactions. It serves as a cornerstone for advancements in fields such as drug discovery, materials science, and catalysis. As we continue to refine our understanding of bond order calculations, we open new avenues for scientific exploration and technological innovation.
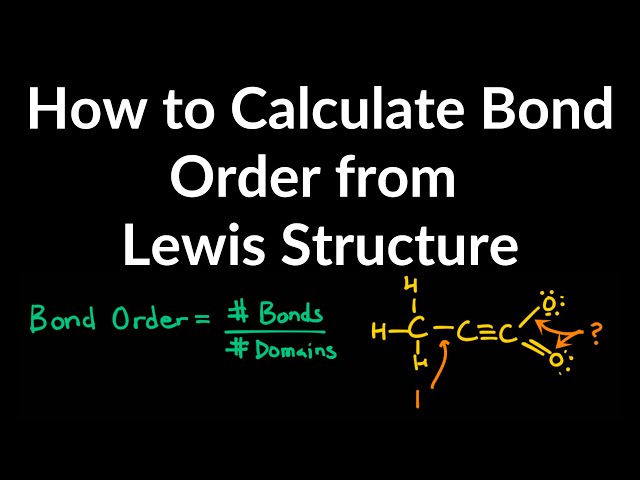