Bond order is a measure of the number of chemical bonds between two atoms. It provides valuable insights into the strength and stability of chemical bonds, which are fundamental to understanding various chemical reactions and molecular structures.
Determining bond order is crucial for comprehending the behavior and properties of molecules. This information aids in predicting reaction pathways and molecular geometry, making it essential in fields such as chemistry, materials science, and biochemistry.
A key historical development in this field was the introduction of the valence bond theory by Linus Pauling in the 1930s. This theory provided a quantum mechanical explanation for bond formation and laid the foundation for understanding bond order.
How to Find Bond Order Between Two Atoms
Determining bond order is crucial for understanding chemical bonding and molecular properties. Key aspects to consider include:
- Atomic number
- Electronegativity
- Hybridization
- Molecular orbital theory
- Bond length
- Bond strength
- Resonance
- Delocalization
These aspects are interconnected and provide a comprehensive understanding of bond order. For instance, atomic number and electronegativity influence the number of valence electrons available for bonding, while hybridization and molecular orbital theory describe the types of orbitals involved in bond formation. Bond length and bond strength are directly related to bond order, and resonance and delocalization can affect the overall bond order in a molecule.
Atomic Number
Atomic number plays a crucial role in determining bond order as it defines the number of protons in the atomic nucleus, which in turn determines the number of electrons in the atom. The number of valence electrons, which are the electrons in the outermost shell, directly influences the bonding behavior of an atom. For example, atoms with a higher atomic number tend to have more valence electrons, making them more likely to form bonds.
In the context of bond order, atomic number affects the number and type of bonds that an atom can form. For instance, atoms with higher atomic numbers tend to form more covalent bonds, where electrons are shared between atoms, as they have more valence electrons available for bonding. Conversely, atoms with lower atomic numbers tend to form more ionic bonds, where one atom donates electrons to another, as they have fewer valence electrons to share.
Understanding the relationship between atomic number and bond order has numerous practical applications. For example, in materials science, it helps in designing materials with specific properties by controlling the types and strengths of bonds formed between atoms. In chemistry, it aids in predicting the reactivity and stability of molecules, as well as in developing new synthetic methods.
Electronegativity
Electronegativity is a crucial aspect in determining bond order between two atoms. It refers to the ability of an atom to attract electrons towards itself in a chemical bond. Electronegativity strongly influences the polarity and strength of chemical bonds, and thus plays a significant role in shaping molecular properties.
- Atomic Number: The number of protons in the nucleus determines an atom’s electronegativity. Higher atomic number generally correlates with higher electronegativity.
- Atomic Radius: Electronegativity decreases with increasing atomic radius. This is because the valence electrons are farther from the nucleus and experience less attraction.
- Electronegativity and Bond Polarity: The difference in electronegativity between two atoms determines the polarity of a bond. A larger difference results in a more polar bond.
- Electronegativity and Bond Strength: Electronegativity affects bond strength. Bonds between atoms with similar electronegativity are typically stronger than those between atoms with large differences in electronegativity.
Understanding electronegativity is crucial for predicting and explaining chemical bonding behavior. It provides insights into molecular polarity, reactivity, and various physical properties. This knowledge is utilized in diverse fields, including materials science, catalysis, and medicinal chemistry, to design and optimize materials and compounds with desired properties.
Hybridization
Hybridization is a fundamental concept in chemistry that describes the process of combining atomic orbitals to form new hybridized orbitals with different shapes and energies. This phenomenon plays a crucial role in determining bond order between two atoms.
Hybridization arises from the interaction of atomic orbitals with similar energies. When atomic orbitals overlap, they can combine to form new orbitals that have different shapes and properties compared to the original atomic orbitals. The type of hybridization that occurs depends on the number and type of atomic orbitals involved in the overlap.
The relationship between hybridization and bond order is evident in the formation of covalent bonds. Covalent bonds are formed when two atoms share electrons in order to achieve a more stable electron configuration. The hybridization of the atomic orbitals involved in the bond determines the geometry and strength of the bond. For example, in a carbon-carbon single bond, the carbon atoms undergo sp3 hybridization, resulting in the formation of four equivalent hybridized orbitals that overlap to form a tetrahedral bond.
Understanding hybridization is critical for accurately determining bond order, as it provides insights into the electronic structure and bonding behavior of molecules. This knowledge is essential in various fields, including chemistry, materials science, and biochemistry, where it aids in predicting molecular properties, designing new materials, and understanding biological processes.
Molecular Orbital Theory
Molecular orbital theory (MOT) is a quantum mechanical approach to describing the electronic structure of molecules. It provides a framework for understanding chemical bonding and various properties of molecules, including bond order. Here are some key aspects of MOT in relation to finding bond order between two atoms:
- Linear Combination of Atomic Orbitals (LCAO): MOT proposes that molecular orbitals are formed by combining atomic orbitals of the constituent atoms. The combination can be either constructive or destructive, leading to bonding or antibonding molecular orbitals.
- Bonding and Antibonding Orbitals: Bonding molecular orbitals result from constructive overlap of atomic orbitals, leading to electron density concentration between the nuclei and a decrease in potential energy. Antibonding molecular orbitals arise from destructive overlap, resulting in electron density depletion between the nuclei and an increase in potential energy.
- Molecular Orbital Energy Levels: The energy levels of molecular orbitals depend on the number and type of atomic orbitals involved in the combination. Bonding orbitals are typically lower in energy than the parent atomic orbitals, while antibonding orbitals are higher in energy.
- Bond Order: Bond order in MOT is determined by the number of electrons occupying bonding molecular orbitals minus the number of electrons in antibonding molecular orbitals. A higher bond order indicates a stronger bond.
MOT provides a powerful tool for understanding the electronic structure and bonding in molecules. By considering the interactions between atomic orbitals and the resulting molecular orbitals, MOT allows for the calculation of bond orders and the prediction of molecular properties.
Bond Length
Bond length is a critical component in determining bond order between two atoms. It refers to the equilibrium distance between the nuclei of two bonded atoms. The relationship between bond length and bond order is inverse, meaning that shorter bond lengths correspond to higher bond orders and vice versa.
The cause of this relationship lies in the strength of the bond. A stronger bond, indicated by a higher bond order, results from a greater overlap between the electron orbitals of the bonded atoms. This increased overlap leads to a decrease in the distance between the nuclei, resulting in a shorter bond length. Conversely, a weaker bond with a lower bond order has less electron overlap and a longer bond length.
Examples of bond length and bond order can be found in various molecules. For instance, in a carbon-carbon single bond, the bond length is typically around 1.54 angstroms, corresponding to a bond order of one. In contrast, a carbon-carbon double bond has a bond length of approximately 1.34 angstroms and a bond order of two. The shorter bond length in the double bond reflects the stronger bond resulting from the greater overlap of electron orbitals.
Understanding the connection between bond length and bond order has practical applications in chemistry and materials science. By manipulating bond lengths, it is possible to tune the properties of materials, such as their strength, conductivity, and reactivity. This knowledge is valuable in designing new materials with specific properties for various technological applications.
Bond Strength
Bond strength is a crucial aspect of determining bond order between two atoms. It refers to the strength of the attractive force that holds atoms together in a chemical bond. The relationship between bond strength and bond order is directly proportional, meaning that stronger bonds have higher bond orders, and vice versa.
The strength of a bond is determined by several factors, including the electronegativity of the atoms involved, the type of bond formed (covalent, ionic, or metallic), and the number of electrons shared between the atoms. Stronger bonds are typically formed between atoms with similar electronegativities and involve the sharing of more electrons. For example, a carbon-carbon single bond is weaker than a carbon-carbon double bond because the latter involves the sharing of four electrons instead of two.
Understanding the relationship between bond strength and bond order has practical applications in various fields, such as chemistry, materials science, and biochemistry. In materials science, for instance, the strength of chemical bonds determines the mechanical properties of materials, such as their strength, ductility, and toughness. In biochemistry, the strength of bonds between atoms within biomolecules influences their stability and function.
Overall, bond strength is an important factor to consider when determining bond order between two atoms. By understanding the relationship between these two concepts, scientists and researchers can gain valuable insights into the properties and behavior of molecules and materials.
Resonance
Resonance is a crucial concept in chemistry that describes the delocalization of electrons within a molecule or ion. It plays a significant role in determining bond order between two atoms, as it can affect the distribution of electrons and the strength of the bonds.
Resonance occurs when there is more than one possible Lewis structure for a molecule or ion. These resonance structures differ in the placement of electrons, but they all have the same number of valence electrons and the same connectivity of atoms. The actual structure of the molecule or ion is a hybrid of all the resonance structures, and the electrons are delocalized over the entire molecule or ion.
The presence of resonance can affect bond order in two ways. First, it can lead to an increase in bond order. This occurs when the resonance structures have alternating double and single bonds between the same two atoms. For example, the carbonate ion (CO32-) has three resonance structures, each with a different double bond between the carbon atom and one of the oxygen atoms. This resonance results in an average bond order of 1.33 between the carbon atom and each oxygen atom, which is higher than the bond order of a typical single bond.
Second, resonance can lead to a decrease in bond order. This occurs when the resonance structures have multiple bonds between different atoms. For example, the benzene molecule (C6H6) has six resonance structures, each with a different arrangement of double bonds between the carbon atoms. This resonance results in an average bond order of 1.5 between each pair of carbon atoms, which is lower than the bond order of a typical double bond.
Understanding resonance is critical for accurately determining bond order between two atoms. By considering the resonance structures of a molecule or ion, chemists can gain insights into the electron distribution and bond strengths within the molecule or ion.
Delocalization
Delocalization refers to the distribution of electrons over several atoms rather than being confined to a specific atom or bond. Understanding delocalization is critical in accurately finding bond order between two atoms, as it can affect the electron distribution and bond strengths within a molecule or ion.
Delocalization can influence bond order in two ways. First, it can lead to an increase in bond order. This occurs when the delocalized electrons contribute to the bonding between two atoms, strengthening the bond. For example, in the benzene molecule (C6H6), the six pi electrons are delocalized over the entire ring, resulting in an average bond order of 1.5 between each pair of carbon atoms, which is higher than the bond order of a typical single bond.
Second, delocalization can lead to a decrease in bond order. This occurs when the delocalized electrons weaken the bond between two atoms. For example, in the allyl anion (CH2=CH-CH2–), the negative charge is delocalized over the three carbon atoms, weakening the bond between the central carbon atom and the carbon atom bearing the negative charge. This results in an average bond order of 1.33 between these two carbon atoms, which is lower than the bond order of a typical single bond.
Understanding delocalization is crucial for accurately determining bond order between two atoms, as it provides insights into the electron distribution and bond strengths within a molecule or ion. This understanding has practical applications in various fields, such as chemistry, materials science, and biochemistry, where it aids in predicting molecular properties, designing new materials, and understanding biological processes.
Frequently Asked Questions (FAQs)
This FAQ section provides answers to commonly asked questions and about finding bond order between two atoms.
Question 1: What is bond order?
Bond order is a measure of the strength of a chemical bond between two atoms. It indicates the number of electron pairs shared between the atoms.
Question 2: How do I determine the bond order between two atoms?
Bond order can be calculated using various methods, including the valence bond theory, molecular orbital theory, and experimental techniques.
Question 3: What factors affect bond order?
Bond order is influenced by factors such as atomic number, electronegativity, hybridization, resonance, and delocalization.
Question 4: What is the relationship between bond order and bond length?
Bond order and bond length are inversely proportional. Stronger bonds with higher bond orders have shorter bond lengths.
Question 5: What is the significance of bond order in chemistry?
Bond order is crucial for understanding molecular structure, stability, reactivity, and various physical and chemical properties.
Question 6: How does bond order help in materials science?
In materials science, understanding bond order aids in designing and engineering materials with desired properties, such as strength, conductivity, and optical behavior.
These FAQs provide a concise overview of key concepts related to finding bond order between two atoms. For a more in-depth discussion, please refer to the comprehensive guide provided in the following section.
Delving into the intricacies of bond order calculations and applications.
Tips for Finding Bond Order Between Two Atoms
This section provides practical tips to assist you in accurately determining bond order between two atoms.
Tip 1: Consider Atomic Number and Electronegativity: Atomic number and electronegativity influence the number and type of bonds an atom can form. A clear understanding of these properties is crucial for predicting bond order.
Tip 2: Determine Hybridization: Hybridization describes the mixing of atomic orbitals to form new hybrid orbitals. Identifying the hybridization of the atoms involved provides insights into the geometry and strength of the bond.
Tip 3: Apply Molecular Orbital Theory: Molecular orbital theory offers a quantum mechanical approach to understanding bond formation. Utilize this theory to calculate bond order and analyze the electronic structure of molecules.
Tip 4: Measure Bond Length: Bond length is inversely related to bond order. Measure or estimate bond lengths to gain insights into the strength of the bond between two atoms.
Tip 5: Analyze Bond Strength: Bond strength is directly proportional to bond order. Conduct experiments or consult databases to determine bond strengths and correlate them to bond orders.
By following these tips, you can enhance your understanding of bond order and its significance in chemistry and materials science.
Moving forward, the concluding section will summarize key takeaways and highlight the implications of bond order in various scientific disciplines.
Conclusion
Throughout this article, we have explored the intricacies of finding bond order between two atoms, examining key concepts such as atomic properties, hybridization, molecular orbital theory, bond length, and bond strength. These factors collectively provide a comprehensive framework for understanding and calculating bond order.
By considering the interplay between these concepts, we gain insights into the fundamental nature of chemical bonding. Bond order serves as a crucial parameter in predicting molecular properties, reactivity, and stability. It plays a significant role in materials science, where tailoring bond order enables the design of materials with specific electronic and structural characteristics.
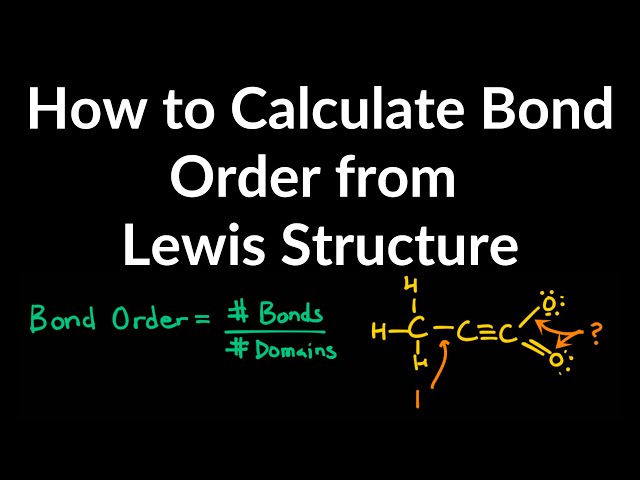