“How bond order is calculated” is a verb phrase that describes a process of determining the number of bonds between two atoms in a molecule.
Understanding bond order is essential for chemists because it helps predict the stability, reactivity, and other properties of molecules. For example, knowing the bond order between carbon and oxygen in carbon monoxide (CO) allows chemists to predict its toxicity and its use as a fuel.
A key historical development in the calculation of bond order was the discovery of quantum mechanics in the early 20th century. This theory allowed chemists to understand the electronic structure of molecules and how it influences bond formation.
how bond order is calculated
Understanding how bond order is calculated is essential for chemists because it provides insights into the nature of the chemical bond and its properties. Here are eight key aspects to consider:
- Atomic number
- Electronegativity
- Bond length
- Bond energy
- Molecular orbital theory
- Hybridization
- Resonance
- Delocalization
These aspects are interconnected and provide a comprehensive understanding of bond order. For example, the atomic number of the atoms involved in the bond determines the number of electrons available for bonding, which in turn influences the bond order. Similarly, the electronegativity of the atoms affects the distribution of electrons in the bond and hence the bond order. By considering these factors, chemists can accurately predict the bond order of a molecule and gain insights into its chemical properties.
Atomic number
Atomic number plays a crucial role in determining bond order. The atomic number of an element represents the number of protons in its nucleus, which in turn determines the number of electrons available for bonding. A higher atomic number generally corresponds to more electrons and a greater potential for bond formation.
- Number of valence electrons
The number of valence electrons, which are the electrons in the outermost shell of an atom, directly affects the bond order. Elements with more valence electrons have a higher potential for forming multiple bonds.
- Electronegativity
Electronegativity measures the ability of an atom to attract electrons towards itself. The difference in electronegativity between two atoms influences the polarity of the bond and the distribution of electrons, which in turn affects the bond order.
- Bond length
The bond length, which is the distance between the nuclei of two bonded atoms, is inversely related to the bond order. A shorter bond length generally indicates a higher bond order and a stronger bond.
- Bond energy
Bond energy is the amount of energy required to break a bond. A higher bond order typically corresponds to a higher bond energy, indicating a more stable bond.
In summary, atomic number provides insights into the number of valence electrons, electronegativity, bond length, and bond energy, all of which are key factors in determining the bond order. Understanding the role of atomic number helps chemists predict the stability and reactivity of molecules, as well as their overall chemical properties.
Electronegativity
Electronegativity is a crucial factor in determining bond order. It measures the ability of an atom to attract electrons towards itself, influencing the polarity of the bond and the distribution of electrons. A higher electronegativity indicates a greater attraction for electrons.
- Atomic number
The atomic number of an element is directly related to its electronegativity. Elements with higher atomic numbers tend to be more electronegative due to the increased number of protons in the nucleus, which attracts electrons more strongly.
- Size of the atom
Smaller atoms have a higher electronegativity because the nucleus is closer to the valence electrons, exerting a stronger attraction.
- Electronegativity and bond polarity
The difference in electronegativity between two atoms determines the polarity of the bond. A larger difference in electronegativity results in a more polar bond, with the more electronegative atom having a partial negative charge and the less electronegative atom having a partial positive charge.
- Electronegativity and bond order
Electronegativity influences bond order by affecting the distribution of electrons in the bond. A more electronegative atom will draw electrons towards itself, resulting in a higher electron density in the bond and a higher bond order.
In summary, electronegativity is a key factor in determining bond order. It affects the polarity of the bond and the distribution of electrons, which in turn influences the bond order. Understanding electronegativity provides insights into the nature of the chemical bond and its properties.
Bond length
Bond length, defined as the equilibrium distance between the nuclei of two bonded atoms, plays a crucial role in determining bond order. A shorter bond length typically indicates a stronger bond and a higher bond order.
- Atomic radii
The atomic radii of the bonded atoms influence the bond length. Atoms with larger atomic radii tend to form longer bonds because their valence electrons are further away from the nucleus.
- Bond order
Bond order is inversely related to bond length. A higher bond order indicates a shorter bond length due to the increased electron density between the atoms.
- Multiple bonds
Multiple bonds, such as double and triple bonds, have shorter bond lengths compared to single bonds between the same atoms. This is because multiple bonds involve more electron pairs shared between the atoms, resulting in a higher electron density and a stronger bond.
- Resonance
Resonance, which occurs when multiple Lewis structures can be drawn for a molecule, affects bond lengths. Resonance structures with shorter bond lengths and higher bond orders are more significant contributors to the overall resonance hybrid.
In conclusion, bond length is an important factor in determining bond order. It provides insights into the strength and nature of the chemical bond, as well as the electronic structure of molecules. Understanding bond length helps chemists predict and rationalize the properties and reactivity of various compounds.
Bond energy
Bond energy, which refers to the amount of energy required to break a chemical bond, is intricately connected to “how bond order is calculated.” The bond order, which represents the number of chemical bonds between two atoms in a molecule, directly influences the bond energy.
A higher bond order typically corresponds to a higher bond energy. This is because a higher bond order indicates a greater number of shared electrons between the atoms, resulting in a stronger bond. Consequently, breaking a bond with a higher bond order requires more energy, leading to a higher bond energy.
The relationship between bond order and bond energy has significant implications in various fields of chemistry. For example, in the context of molecular stability, compounds with stronger bonds (higher bond orders and bond energies) are generally more stable and less reactive. Additionally, understanding the bond order and bond energy of a molecule can provide insights into its chemical reactivity and reaction pathways.
In conclusion, bond energy is a critical component of “how bond order is calculated.” The bond order and bond energy are directly related, with a higher bond order typically corresponding to a higher bond energy. This understanding is essential for predicting the stability, reactivity, and other properties of chemical compounds.
Molecular orbital theory
Molecular orbital theory (MOT) is a fundamental concept in quantum chemistry that describes the electronic structure of molecules. It provides a mathematical framework for understanding how electrons are distributed within a molecule and how this distribution determines the chemical bonding between atoms.
MOT is critical for calculating bond order because it provides a way to determine the number of electrons that are shared between two atoms. The bond order, in turn, is a measure of the strength of the bond. A higher bond order indicates a stronger bond. MOT can also be used to explain other properties of molecules, such as their stability, reactivity, and magnetic properties.
One of the most important applications of MOT is in the design of new materials. By understanding how the electronic structure of a molecule affects its properties, chemists can design materials with specific properties, such as high strength, low thermal conductivity, or high electrical conductivity.
In conclusion, MOT is a powerful tool that can be used to understand the electronic structure of molecules and predict their properties. It is essential for calculating bond order and has a wide range of applications in chemistry, including the design of new materials.
Hybridization
Hybridization is a fundamental concept in chemistry that describes the mixing of atomic orbitals to form new hybrid orbitals with different shapes and energies. It is a crucial aspect of “how bond order is calculated” because the type of hybridization of the atomic orbitals involved in a bond determines the bond’s strength and properties.
- Types of Hybridization
There are four main types of hybridization: sp, sp2, sp3, and sp3d. Each type of hybridization results in a different number of hybrid orbitals with specific shapes and orientations.
- Bond Strength
The strength of a bond is directly related to the type of hybridization of the atomic orbitals involved. Bonds formed from hybrid orbitals with more p-character are generally stronger than those formed from hybrid orbitals with more s-character.
- Bond Angle
The bond angle between two atoms is also affected by the hybridization of their atomic orbitals. Hybrid orbitals with more p-character tend to form bonds with smaller bond angles than those with more s-character.
In conclusion, hybridization is a key factor in determining bond order because it affects the type of bond that is formed, the strength of the bond, and the bond angle. Understanding hybridization is therefore essential for accurately calculating bond order and predicting the properties of molecules.
Resonance
Resonance is a crucial concept in chemistry that helps explain the bonding and properties of certain molecules. It plays a significant role in “how bond order is calculated” because it allows chemists to represent the delocalization of electrons in a molecule using multiple Lewis structures.
- Delocalized Electrons
Resonance structures show the delocalization of electrons, meaning that the electrons are not confined to a specific bond or atom. This delocalization can occur when there are multiple equivalent positions for electrons within a molecule.
- Equivalent Lewis Structures
Resonance structures are different Lewis structures that represent the same molecule. They have the same number of atoms and electrons, but the arrangement of the electrons differs. The resonance hybrid is a weighted average of the resonance structures, and it best represents the actual electronic structure of the molecule.
- Bond Order
The bond order of a bond is determined by the number of electrons that are shared between the two atoms involved in the bond. In resonance structures, the bond order of a bond can vary depending on the resonance structure. The overall bond order of the molecule is the average of the bond orders in the resonance structures.
- Stability
Resonance can contribute to the stability of a molecule. Molecules with resonance structures are generally more stable than molecules without resonance structures. This is because the delocalization of electrons lowers the overall energy of the molecule.
In conclusion, resonance is an important concept in chemistry that helps explain the bonding and properties of certain molecules. It plays a significant role in “how bond order is calculated” because it allows chemists to represent the delocalization of electrons in a molecule using multiple Lewis structures. This delocalization can affect the bond order and stability of the molecule.
Delocalization
Delocalization is a crucial aspect of “how bond order is calculated” because it allows electrons to be shared over multiple atoms, affecting the strength and properties of the bonds. This delocalization can occur in various ways.
- Resonance
Resonance is a phenomenon where multiple Lewis structures can be drawn for a molecule, with the actual structure being a hybrid of these resonance structures. Delocalization of electrons occurs when there is more than one possible Lewis structure for a molecule.
- Aromatic Rings
Aromatic rings are cyclic structures with alternating single and double bonds. The electrons in the pi orbitals of the double bonds are delocalized over the entire ring, resulting in increased stability.
- Metal-Ligand Bonding
In metal-ligand complexes, the electrons in the ligand orbitals can delocalize into the metal orbitals. This delocalization can strengthen the bond between the metal and the ligand.
Delocalization has a significant impact on “how bond order is calculated”. Resonance structures, aromatic rings, and metal-ligand bonding all contribute to the delocalization of electrons in a molecule. This delocalization can lower the overall energy of the molecule, making it more stable. It can also affect the bond order of individual bonds, as the electrons are shared over multiple atoms.
Frequently Asked Questions
This FAQ section provides concise answers to commonly asked questions and clarifies essential aspects of “how bond order is calculated”.
Question 1: What is bond order and why is it important?
Answer: Bond order is a measure of the strength of a chemical bond, indicating the number of electron pairs shared between two atoms. It is crucial for understanding molecular structure and predicting chemical reactivity.
Question 2: How is bond order calculated using the Lewis structure?
Answer: Bond order equals half the difference between the number of valence electrons in the atoms involved (total valence electrons) and the number of non-bonding electrons (lone pairs).
Question 3: What factors affect bond order?
Answer: Factors influencing bond order include atomic number, electronegativity, resonance, hybridization, and delocalization of electrons.
Question 4: How is bond order related to bond length and bond energy?
Answer: Bond order is inversely proportional to bond length (shorter bonds have higher bond order) and directly proportional to bond energy (higher bond order corresponds to stronger bonds).
Question 5: Can bond order be used to predict the stability of a molecule?
Answer: Yes, molecules with higher bond orders are generally more stable due to lower overall energy.
Question 6: What are the limitations of using bond order to describe chemical bonding?
Answer: Bond order is a simplified representation of bonding and may not accurately reflect the complex behavior of electrons in molecules, especially in cases involving resonance and delocalization.
In summary, understanding “how bond order is calculated” provides valuable insights into the nature of chemical bonds and their influence on molecular properties. The concept of bond order is a fundamental tool for chemists to analyze and predict the behavior of molecules.
In the next section, we will delve deeper into advanced methods for calculating bond order, considering factors such as resonance and delocalization, to gain a more comprehensive understanding of molecular bonding.
Tips for Calculating Bond Order
This section provides practical tips to enhance your understanding and accuracy when calculating bond order.
Tip 1: Determine the total number of valence electrons involved in the bond by summing the valence electrons of the bonded atoms.
Tip 2: Count the number of non-bonding electrons (lone pairs) on each atom and subtract this value from the total valence electrons.
Tip 3: Divide the resulting value by 2 to obtain the bond order. A bond order of 1 indicates a single bond, 2 indicates a double bond, and so on.
Tip 4: Consider resonance structures when calculating bond order. If resonance occurs, the bond order is the average of the bond orders in all contributing resonance structures.
Tip 5: Understand the relationship between bond order and bond properties. Higher bond order generally corresponds to shorter bond lengths and stronger bond energies.
Tip 6: Utilize molecular orbital theory to gain insights into the electronic structure of the molecule and its impact on bond order.
Tip 7:
Tip 8: Employ computational chemistry methods, such as density functional theory (DFT), to calculate bond orders with greater accuracy, especially for complex molecules.
By following these tips, you can effectively calculate bond orders and gain a deeper understanding of chemical bonding.
In the concluding section, we will explore advanced concepts related to bond order, including resonance, delocalization, and molecular orbital theory, to further enhance your knowledge of chemical bonding.
Conclusion
In summary, understanding “how bond order is calculated” provides invaluable insights into the nature of chemical bonding and its influence on molecular properties. We have explored the fundamental concepts of bond order, examining factors such as atomic number, electronegativity, resonance, hybridization, and delocalization.
The calculation of bond order is not merely a numerical exercise but a powerful tool for understanding and predicting the behavior of molecules. By considering the various factors that influence bond order, chemists can gain insights into molecular stability, reactivity, and other important properties.
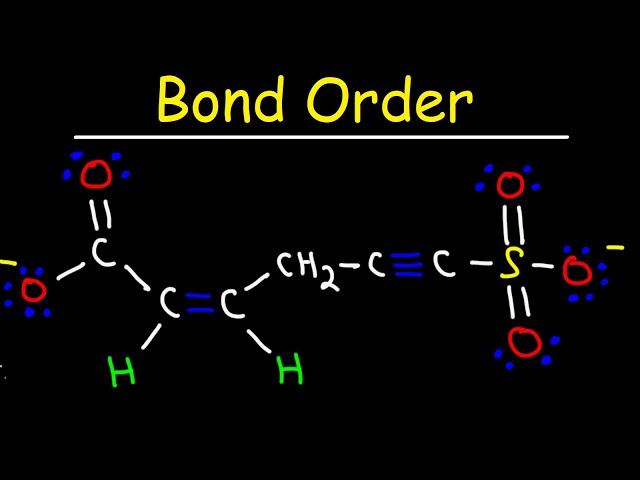