Effective bond order, defined as a quantitative measure of bond strength, is essential in predicting the reactivity and stability of chemical compounds. Its determination plays a pivotal role in various scientific fields, including chemistry, materials science, and biochemistry.
The concept of effective bond order emerged as a result of advancements in quantum mechanics. By calculating the electron density between atoms, scientists can determine the strength of the bond between them. These techniques, pioneered by Linus Pauling and others, have revolutionized our understanding of chemical bonding.
This article delves into the intricacies of determining effective bond order, exploring its various methods, applications, and implications. We will examine established techniques, recent developments, and the significance of this concept for scientific research and technological advancements.
How to Find Effective Bond Order
Determining effective bond order is essential for understanding chemical bonding and predicting the properties of molecules. Key aspects to consider include:
- Electron density
- Bond length
- Bond strength
- Resonance
- Hybridization
- Atomic orbitals
- Molecular geometry
- Electronegativity
These aspects are interconnected and provide a comprehensive understanding of bond order. Electron density, for example, is inversely related to bond length and directly related to bond strength. Resonance and hybridization can influence bond order by delocalizing electrons and modifying atomic orbital overlap. Additionally, molecular geometry and electronegativity affect bond order by influencing the distribution of electrons within the molecule.
Electron density
Electron density is a fundamental concept in chemistry that plays a crucial role in determining effective bond order. It refers to the probability of finding an electron within a specific region of space around an atom or molecule.
- Overlap
Electron density is highest in regions where atomic orbitals overlap. The greater the overlap, the stronger the bond. - Bond length
Electron density is inversely proportional to bond length. As the bond length increases, the electron density between the atoms decreases, resulting in a weaker bond. - Electronegativity
Electronegativity is a measure of an atom’s ability to attract electrons. The more electronegative an atom, the higher the electron density around it. - Resonance
Resonance occurs when multiple Lewis structures can be drawn for a molecule. This delocalization of electrons increases the electron density in certain regions of the molecule, leading to stronger bonds.
Understanding electron density is essential for predicting bond order and understanding the behavior of molecules. It provides valuable insights into the strength, length, and polarity of chemical bonds, enabling chemists to design and synthesize new materials with desired properties.
Bond length
Bond length is a critical component of how to find effective bond order. It refers to the distance between the nuclei of two bonded atoms and is inversely proportional to bond order. The shorter the bond length, the stronger the bond and the higher the bond order.
Bond length is determined by several factors, including the atomic radii of the bonded atoms, the number of bonding electrons, and the hybridization of the atomic orbitals involved in the bond. For example, a carbon-carbon single bond has a longer bond length than a carbon-carbon double bond because the double bond has more bonding electrons and shorter bond length due to increased overlap of atomic orbitals.
Understanding bond length is essential for predicting the properties of molecules and materials. For example, shorter bond lengths typically result in stronger bonds, higher bond dissociation energies, and increased chemical reactivity. This knowledge is applied in fields such as materials science, where the design of new materials with specific properties requires precise control over bond lengths.
Bond strength
Bond strength is a fundamental property of chemical bonds that directly influences the behavior and properties of molecules. Understanding bond strength is crucial for determining effective bond order, which provides valuable insights into the nature of chemical bonding.
Bond strength is primarily determined by the number of bonding electrons, the overlap of atomic orbitals, and the electronegativity of the bonded atoms. Stronger bonds are formed when there are more bonding electrons, greater overlap of atomic orbitals, and less difference in electronegativity between the bonded atoms.
Real-life examples of bond strength can be found in various chemical compounds and materials. For instance, the strong covalent bonds in diamond contribute to its exceptional hardness and thermal conductivity. In contrast, the weaker hydrogen bonds in water allow for its unique properties, such as high surface tension and the ability to dissolve many substances.
Understanding bond strength has practical applications in diverse fields. In materials science, it guides the design and synthesis of materials with desired properties, such as strength, durability, and thermal stability. In biochemistry, it helps elucidate the structure and function of biological molecules, including proteins and DNA.
Resonance
Resonance is a fundamental aspect of Lewis theory that plays a crucial role in determining effective bond order. It arises when multiple valid Lewis structures can be drawn for a molecule, indicating that the bonding electrons are delocalized over several atomic centers.
- Equivalent Resonance Structures
Resonance structures have the same number of valence electrons and obey all the rules of Lewis structures. They differ only in the placement of double and single bonds.
- Delocalized Electrons
In resonance structures, the electrons involved in multiple bonds are not confined to a specific bond but are spread out over the entire molecule.
- Bond Order
Resonance affects bond order by increasing the electron density in certain regions of the molecule. This leads to an increase in bond order for the bonds involved in resonance.
- Stability
Resonance contributes to the stability of molecules by lowering their overall energy. Molecules with more resonance structures are generally more stable.
Understanding resonance is essential for accurately determining effective bond order and predicting the properties of molecules. It provides valuable insights into the electronic structure and bonding behavior of molecules, enabling chemists to design and synthesize new materials with desired functionalities.
Hybridization
Hybridization is a fundamental concept in chemistry that describes the mixing of atomic orbitals to form new hybrid orbitals with different shapes and energies. It plays a crucial role in determining effective bond order, as the type of hybridization influences the overlap of atomic orbitals and the strength of the resulting chemical bond.
Hybridization affects bond order by altering the number and type of atomic orbitals that participate in bonding. For example, in a carbon atom, the 2s and three 2p orbitals can hybridize to form four equivalent sp3 hybrid orbitals. These hybrid orbitals have a tetrahedral geometry and allow the carbon atom to form four strong sigma bonds with other atoms. The effective bond order for each of these bonds is one, indicating a single bond.
Understanding hybridization is essential for accurately predicting bond order and the properties of molecules. It provides valuable insights into the electronic structure and bonding behavior of atoms, enabling chemists to design and synthesize new materials with desired functionalities. For instance, in the field of materials science, hybridization is used to control the electronic and optical properties of semiconductors and other materials.
Atomic orbitals
Atomic orbitals are fundamental to understanding how to find effective bond order. They describe the three-dimensional space around an atom where electrons are most likely to be found. The type of atomic orbitals involved in bonding, such as s, p, or d orbitals, determines the shape and strength of the resulting chemical bond.
The overlap of atomic orbitals is critical for bond formation. When atomic orbitals overlap, electrons can be shared between atoms, leading to the formation of a chemical bond. The extent of overlap determines the strength of the bond. For example, head-to-head overlap of two p orbitals results in a strong sigma bond, while lateral overlap of two p orbitals results in a weaker pi bond.
Understanding atomic orbitals is essential for predicting the properties of molecules and materials. By analyzing the atomic orbitals involved in bonding, chemists can determine the bond order, bond length, and bond strength. This knowledge is applied in various fields, including materials science, where the design of new materials with specific properties requires precise control over the atomic orbitals involved in bonding.
Molecular geometry
Molecular geometry refers to the three-dimensional arrangement of atoms within a molecule. It is a crucial component of how to find effective bond order, as it directly influences the overlap of atomic orbitals and, consequently, the strength and type of chemical bonds formed.
The relationship between molecular geometry and effective bond order is bidirectional. On one hand, molecular geometry determines the effective bond order by dictating the number and type of atomic orbitals that overlap. For instance, in a linear molecule with sp hybridization, the atomic orbitals overlap head-to-head, resulting in a strong sigma bond with a bond order of one. Conversely, in a trigonal planar molecule with sp2 hybridization, the atomic orbitals overlap laterally, leading to weaker pi bonds with a bond order of 0.5.
Conversely, effective bond order can influence molecular geometry. For example, in a molecule with resonance, the delocalization of electrons leads to an increase in bond order, which can affect the molecular geometry. This is because the increased bond order reduces the bond length and alters the angles between the bonds.
Understanding the connection between molecular geometry and effective bond order is essential for predicting the properties and reactivity of molecules. It finds applications in various fields, including materials science, where the design of new materials with specific properties requires precise control over molecular geometry and effective bond order.
Electronegativity
Electronegativity is a crucial property of atoms that significantly influences how to find effective bond order. It measures the ability of an atom to attract electrons towards itself within a chemical bond. The electronegativity of an atom is directly related to its atomic number and the number of valence electrons it possesses.
A higher electronegativity difference between two bonded atoms leads to a more polar bond, where the electrons are drawn towards the more electronegative atom. This polarization affects the electron density distribution within the bond, influencing the effective bond order. For instance, in a bond between carbon and oxygen, oxygen’s higher electronegativity attracts electrons towards itself, resulting in a polar bond with a higher effective bond order than a non-polar carbon-carbon bond.
Understanding the relationship between electronegativity and effective bond order is essential for predicting molecular properties and reactivity. Electronegativity is a critical component of various computational methods used to determine effective bond order, such as the electronegativity equalization method (EEM). This understanding finds practical applications in fields like materials science and drug design, where precise control over bond order is crucial for tailoring material properties and optimizing drug efficacy.
Frequently Asked Questions on Finding Effective Bond Order
This section addresses common questions and clarifies aspects of how to find effective bond order, providing concise answers to guide your understanding.
Question 1: What is the significance of effective bond order?
Answer: Effective bond order quantifies bond strength and provides insights into molecular stability, reactivity, and properties. It helps predict chemical behavior and design materials with tailored properties.
Question 2: How is effective bond order calculated?
Answer: Various methods exist, including the Wiberg bond index, natural bond orbital analysis, and density functional theory. Each method utilizes different approaches to determine the electron density distribution and calculate bond order.
Question 3: What factors influence effective bond order?
Answer: Factors include bond length, bond strength, resonance, hybridization, atomic orbital overlap, electronegativity, and molecular geometry. Understanding these factors is crucial for accurate bond order determination.
Question 4: How can effective bond order be used in practice?
Answer: Effective bond order finds applications in diverse fields, such as materials science, drug design, and quantum chemistry. It aids in predicting material properties, optimizing drug efficacy, and understanding chemical reactions.
Question 5: What are the limitations of effective bond order?
Answer: It’s important to note that effective bond order is a theoretical concept and may not always perfectly reflect experimental observations. Factors like temperature, solvent effects, and relativistic effects can influence bond order.
Question 6: How does effective bond order relate to molecular properties?
Answer: Effective bond order correlates with various molecular properties, including bond length, bond dissociation energy, vibrational frequencies, and molecular stability. Understanding these relationships is essential for predicting and manipulating molecular behavior.
These FAQs provide key insights into the concept of effective bond order, its calculation methods, influencing factors, applications, limitations, and connections to molecular properties. Delving further into the topic, the subsequent section explores advanced aspects of effective bond order determination and its significance in chemical research.
Tips for Determining Effective Bond Order
To enhance your understanding of effective bond order determination, consider these practical tips:
Tip 1: Understand the underlying concepts: Grasp the fundamentals of bond order, electron density, and orbital interactions to lay a solid foundation for accurate calculations.
Tip 2: Select appropriate methods: Choose the bond order calculation method that aligns with the accuracy and computational resources required for your specific research question.
Tip 3: Analyze contributing factors: Identify and assess the factors influencing bond order, including bond length, hybridization, resonance, and electronegativity, to gain a comprehensive understanding of the bonding interactions.
Tip 4: Utilize computational tools: Leverage computational chemistry software and online resources to perform complex bond order calculations efficiently and accurately.
Tip 5: Validate your results: Compare your calculated bond order values with experimental data or theoretical estimates to ensure the reliability of your findings.
Tip 6: Consider relativistic effects: For heavy atoms and systems involving strong magnetic fields, account for relativistic effects that can influence bond order.
In summary, these tips empower you to effectively determine bond order, providing valuable insights into molecular structure, bonding characteristics, and chemical reactivity.
Building upon this foundation, the concluding section delves into advanced applications of effective bond order determination, exploring its significance in modern chemical research.
Conclusion
This article has provided a comprehensive exploration of “how to find effective bond order,” shedding light on its significance in understanding chemical bonding and predicting molecular properties. Key insights include the influence of electron density, bond length, and resonance on bond order, as well as the role of hybridization, atomic orbitals, and electronegativity in shaping molecular geometry and bonding interactions.
Understanding effective bond order empowers chemists to design and synthesize new materials with tailored properties, optimize drug efficacy, and unravel the complexities of chemical reactions. It serves as a fundamental tool in diverse fields, including materials science, drug discovery, and quantum chemistry, enabling researchers to probe the intricate world of molecular interactions.
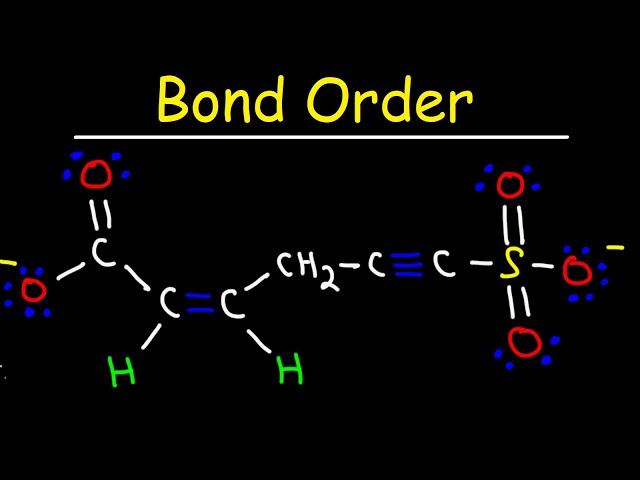