Understanding the bond order of ozone (O3) is essential for chemistry students, environmental scientists, and those in related fields. It helps predict molecular properties and explains the behavior of ozone in the atmosphere and various chemical reactions.
The concept of bond order was first introduced by Linus Pauling in the 1930s. It’s a measure of the number of chemical bonds between two atoms and plays a role in determining the stability and reactivity of a molecule.
This article delves into the steps involved in calculating the bond order of O3, exploring its significance in understanding molecular structure and properties.
How to Calculate the Bond Order of O3
Determining the bond order of ozone (O3) provides insights into its molecular structure and properties. Here are nine key aspects to consider:
- Atomic orbitals
- Molecular orbitals
- Bonding electrons
- Antibonding electrons
- Bond length
- Bond strength
- Molecular stability
- Chemical reactivity
- Spectroscopic properties
Understanding these aspects enables us to predict the behavior of O3 in various chemical reactions and its role in atmospheric chemistry, ozone depletion, and environmental science.
Atomic orbitals
Atomic orbitals are fundamental to understanding how to calculate the bond order of O3. They describe the three-dimensional space around an atom where electrons are most likely to be found. The overlap of atomic orbitals between two atoms leads to the formation of molecular orbitals, which dictate the bonding and antibonding interactions within a molecule.
In the case of O3, the atomic orbitals of the oxygen atoms overlap to form molecular orbitals. These molecular orbitals can be classified as bonding or antibonding based on their symmetry and how they contribute to the overall bond strength of the molecule. The bond order of O3 is determined by the number of bonding electrons (those that occupy bonding molecular orbitals) minus the number of antibonding electrons.
By considering the atomic orbitals involved in the bonding of O3, chemists can gain insights into the molecule’s stability, reactivity, and spectroscopic properties. This understanding has practical applications in fields such as atmospheric chemistry, materials science, and environmental science.
Molecular orbitals
Understanding molecular orbitals is crucial for calculating the bond order of ozone (O3). Molecular orbitals describe the behavior of electrons within a molecule and provide insights into the bonding interactions between atoms.
- Electron density: Molecular orbitals depict the regions where electrons are most likely to be found, helping us visualize the electron distribution within the O3 molecule.
- Bonding/Antibonding: Molecular orbitals can be classified as bonding (stabilizing) or antibonding (destabilizing), indicating their contribution to the overall bond strength of O3.
- Symmetry: The symmetry of molecular orbitals influences their overlap and bonding characteristics, affecting the stability and properties of O3.
- Energy levels: The energy levels of molecular orbitals determine the wavelengths of light absorbed or emitted by O3, providing information about its electronic transitions and spectroscopic properties.
By analyzing the molecular orbitals of O3, chemists can gain a comprehensive understanding of its electronic structure, bonding, and behavior. This knowledge is essential for predicting the molecule’s reactivity, stability, and interactions with other species, with applications in atmospheric chemistry, materials science, and various other fields.
Bonding electrons
Bonding electrons are a crucial aspect in understanding how to calculate the bond order of ozone (O3). These are the electrons that participate in the formation of chemical bonds between atoms, determining the stability and properties of the molecule.
- Number of Bonding Electrons: The number of bonding electrons directly influences the bond order, with a higher number indicating a stronger bond. In O3, the bonding electrons are responsible for holding the three oxygen atoms together.
- Electron Delocalization: Bonding electrons in O3 are delocalized, meaning they are not confined to a specific pair of atoms but rather distributed over the entire molecule. This delocalization contributes to the stability of O3.
- Bond Length: The bond order is inversely related to the bond length. As the bond order increases, the bond length decreases, indicating a stronger and shorter bond between the atoms.
- Reactivity: The number of bonding electrons influences the reactivity of O3. Ozone with a higher bond order is less reactive as the electrons are more strongly held within the molecule.
Understanding the role of bonding electrons in O3 provides valuable insights into its molecular structure, properties, and behavior. These concepts are essential for predicting the reactivity, stability, and spectroscopic characteristics of O3, which have implications in atmospheric chemistry, materials science, and other fields.
Antibonding Electrons
Antibonding electrons play a crucial role in the calculation of the bond order of ozone (O3). The bond order, a measure of the strength of the chemical bond between atoms, is influenced by both bonding electrons (those that contribute to the formation of the bond) and antibonding electrons (those that weaken the bond).
In O3, the antibonding electrons occupy molecular orbitals that have a nodal plane between the bonded atoms. This nodal plane creates a region of zero electron density, reducing the overlap between the atomic orbitals and weakening the bond. The number of antibonding electrons affects the overall bond order, with a higher number of antibonding electrons resulting in a lower bond order and a weaker bond.
Understanding the concept of antibonding electrons is essential for accurately calculating the bond order of O3 and predicting its properties. For example, O3 with a lower bond order is more reactive and more likely to participate in chemical reactions. This understanding has practical applications in fields such as atmospheric chemistry, where ozone plays a crucial role in protecting the Earth from harmful UV radiation.
Bond length
Bond length is a fundamental aspect in understanding how to calculate the bond order of ozone (O3). It plays a crucial role in determining the stability and properties of the molecule.
- Bond Strength:
Bond length is inversely proportional to bond strength. A shorter bond length indicates a stronger bond between the atoms.
- Resonance Structures:
In O3, the resonance structures contribute to the equalization of bond lengths, resulting in an average bond length.
- Atomic Radii:
The bond length is influenced by the atomic radii of the bonded atoms. Larger atoms generally form longer bonds.
Understanding the concept of bond length provides valuable insights into the molecular structure and properties of O3. It helps predict the reactivity, stability, and spectroscopic characteristics of the molecule, which have implications in atmospheric chemistry, materials science, and other fields.
Bond strength
Bond strength is a crucial aspect in calculating the bond order of ozone (O3). It provides insights into the stability and reactivity of the molecule, influencing its behavior in various chemical processes and atmospheric phenomena.
- Bond Enthalpy:
Bond enthalpy measures the energy required to break a bond. A higher bond enthalpy indicates a stronger bond and a more stable molecule of O3.
- Bond Length:
Bond strength is inversely proportional to bond length. Stronger bonds are typically shorter, as the atoms are held more tightly together in the O3 molecule.
- Electronegativity:
Electronegativity influences bond strength. Atoms with higher electronegativity attract electrons more strongly, resulting in stronger bonds in O3.
- Resonance:
Resonance structures contribute to bond strength in O3. By delocalizing electrons, resonance stabilizes the molecule and strengthens the overall bond.
Understanding bond strength is essential for predicting the reactivity, stability, and spectroscopic properties of O3. Stronger bonds indicate a more stable molecule, less likely to undergo chemical reactions. This knowledge is vital in fields such as atmospheric chemistry, where ozone plays a critical role in protecting the Earth from harmful UV radiation.
Molecular Stability
Understanding molecular stability is crucial in calculating the bond order of ozone (O3), as it reflects the molecule’s resistance to decomposition or change. The bond order, a measure of bond strength, directly influences the stability of O3.
A higher bond order indicates stronger bonds between the oxygen atoms in O3, leading to increased molecular stability. This stability is essential for ozone to perform its vital role in the Earth’s atmosphere, absorbing harmful ultraviolet radiation. Conversely, a lower bond order results in weaker bonds and reduced stability, making O3 more susceptible to decomposition.
Calculating the bond order of O3 provides insights into its stability, allowing scientists to predict its behavior and reactivity in various environments. This understanding has practical applications in atmospheric chemistry, where ozone depletion and formation are critical concerns. By manipulating bond order through chemical reactions or external factors, scientists can potentially influence ozone levels and mitigate environmental impacts.
Chemical Reactivity
Chemical reactivity is a crucial aspect in understanding how to calculate the bond order of ozone (O3). It reflects the tendency of O3 to undergo chemical reactions and interact with other molecules. The bond order, a measure of bond strength and stability, directly influences the chemical reactivity of O3.
A higher bond order typically indicates decreased chemical reactivity. Stronger bonds within the O3 molecule make it less likely to participate in chemical reactions because more energy is required to break the bonds. Conversely, a lower bond order suggests increased chemical reactivity. Weaker bonds make the O3 molecule more susceptible to reactions, as the bonds can be broken more easily.
Calculating the bond order of O3 provides valuable insights into its reactivity. By understanding the bond order, scientists can predict the behavior of O3 in various environments and chemical processes. This knowledge has practical applications in atmospheric chemistry, where ozone plays a critical role in absorbing harmful ultraviolet radiation from the sun. By manipulating the bond order through chemical reactions or external factors, scientists can potentially influence ozone levels and mitigate environmental impacts.
Spectroscopic properties
Spectroscopic properties play a crucial role in determining the bond order of ozone (O3). The interaction of light with O3 molecules provides valuable information about their electronic structure and bonding characteristics.
When O3 molecules absorb light at specific wavelengths, they undergo electronic transitions. These transitions correspond to the excitation of electrons from one energy level to another. By analyzing the wavelengths of light absorbed or emitted by O3, scientists can identify the energy differences between different molecular orbitals.
The energy difference between molecular orbitals is directly related to the bond order. Higher bond orders result in larger energy differences, leading to absorption or emission of light at higher frequencies (shorter wavelengths). Conversely, lower bond orders correspond to smaller energy differences and absorption or emission of light at lower frequencies (longer wavelengths).
Practical applications of this understanding include the use of UV-visible spectroscopy to determine the concentration of O3 in the atmosphere. By measuring the absorbance of light at specific wavelengths, scientists can quantify the amount of O3 present and monitor changes in ozone levels over time.
FAQs on Calculating the Bond Order of O3
This section aims to address frequently asked questions and clarify essential aspects of calculating the bond order of ozone (O3) for a comprehensive understanding.
Question 1: What is the significance of bond order in O3?
Answer: Bond order determines the strength and stability of the chemical bond between oxygen atoms in O3, influencing its reactivity and spectroscopic properties.
Question 2: How do I determine the bond order of O3?
Answer: The bond order can be calculated using molecular orbital theory, considering the number of bonding and antibonding electrons in the O3 molecule.
Question 3: What factors affect the bond order of O3?
Answer: Factors such as atomic orbital overlap, electron delocalization, and resonance contribute to the bond order and overall stability of O3.
Question 4: How does bond order relate to bond length and bond strength?
Answer: Bond order is inversely proportional to bond length and directly proportional to bond strength. A higher bond order indicates a shorter and stronger bond.
Question 5: What are the applications of understanding bond order in O3?
Answer: Insights into the bond order of O3 aid in predicting its reactivity, stability, and spectroscopic properties, which have implications in atmospheric chemistry, materials science, and environmental monitoring.
Question 6: How does resonance affect the bond order of O3?
Answer: Resonance delocalizes electrons, contributing to the equalization of bond lengths and an overall higher bond order in O3.
These FAQs provide a concise overview of the key concepts related to calculating the bond order of O3. Understanding these concepts is essential for a deeper exploration of the molecule’s properties and its significance in various scientific fields.
The following section delves into the experimental techniques employed to determine the bond order of O3, offering further insights into the practical applications of this knowledge.
Tips for Calculating the Bond Order of O3
Understanding the intricacies of bond order calculations for O3 requires a combination of theoretical knowledge and practical techniques. Here are five essential tips to guide you through the process effectively:
Tip 1: Grasp Molecular Orbital Theory: Delve into the fundamental principles of molecular orbital theory to comprehend the concepts of bonding and antibonding electrons, crucial for determining bond order.
Tip 2: Count Bonding and Antibonding Electrons: Accurately identify the number of electrons occupying bonding and antibonding molecular orbitals. The difference between these counts directly determines the bond order.
Tip 3: Consider Resonance Structures: Recognize the resonance structures of O3, which contribute to the delocalization of electrons and influence the overall bond order.
Tip 4: Utilize Computational Tools: Employ computational chemistry software or online tools specifically designed for bond order calculations to obtain precise results.
Tip 5: Validate Results Experimentally: Corroborate your calculated bond order with experimental data obtained from spectroscopic techniques, such as UV-visible spectroscopy or IR spectroscopy.
By adhering to these tips, you can confidently navigate the nuances of bond order calculations for O3, gaining valuable insights into its molecular structure and properties.
These tips lay the foundation for exploring advanced concepts and applications related to bond order in O3, as discussed in the subsequent sections of this article.
Conclusion
The exploration of “how to calculate the bond order of O3” unveiled key concepts and their interconnections, providing a comprehensive understanding of this crucial molecular property. Firstly, grasping molecular orbital theory and accurately counting bonding and antibonding electrons are essential for determining bond order. Secondly, recognizing resonance structures contributes to understanding the delocalization of electrons and its impact on bond order.
Comprehending these principles enables chemists to predict the behavior and reactivity of O3 in various chemical and atmospheric processes. By harnessing computational tools and validating results experimentally, scientists can obtain precise bond order values, leading to advancements in fields such as atmospheric chemistry, materials science, and environmental monitoring.
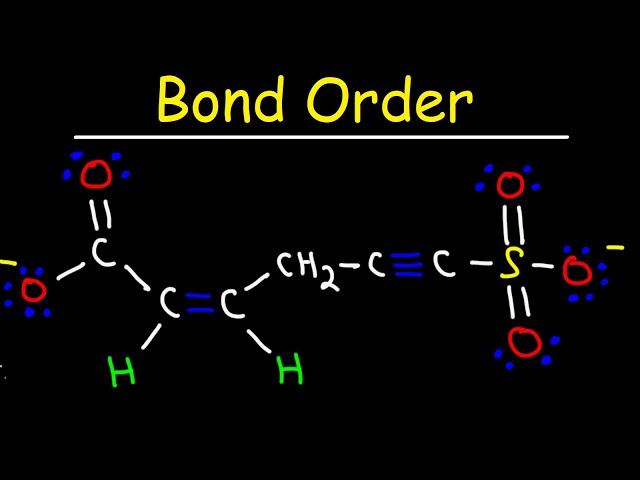