Calculating the bond order of carbon dioxide (CO2) is a crucial step in understanding its chemical behavior. Bond order refers to the number of chemical bonds between two atoms and directly affects a molecule’s stability, reactivity, and physical properties.
Determining bond order helps us comprehend molecular bonding, predict molecular geometry, and assess the strength and polarity of chemical bonds. The concept was first developed by Linus Pauling in the 1930s and has since become a cornerstone of chemistry.
This article will delve into the methods for calculating the bond order of CO2, exploring the underlying principles and providing practical examples to enhance your understanding of this fundamental chemical concept.
How to Calculate the Bond Order of CO2
Calculating the bond order of carbon dioxide (CO2) is a crucial aspect of understanding its chemical behavior. The bond order, representing the number of chemical bonds between two atoms, significantly influences a molecule’s stability, reactivity, and physical properties.
- Molecular Orbital Theory
- Hybridization
- Resonance
- Electronegativity
- Bond Length
- Bond Energy
- Magnetic Properties
- Spectroscopic Properties
These key aspects provide a multifaceted understanding of bond order calculations. Molecular orbital theory explains the formation of molecular orbitals and their role in determining bond order. Hybridization describes the mixing of atomic orbitals to form new hybrid orbitals, affecting bond order. Resonance involves the delocalization of electrons, influencing bond order distribution. Electronegativity, bond length, and bond energy are interrelated factors that impact bond order. Magnetic and spectroscopic properties provide experimental evidence for bond order determination.
Molecular Orbital Theory
Molecular orbital theory (MOT) is a fundamental concept in quantum chemistry that provides a framework for understanding and calculating the bond order of molecules. It involves the combination of atomic orbitals to form molecular orbitals, which describe the distribution of electrons within a molecule.
- Atomic Orbitals
Atomic orbitals are the wave functions that describe the behavior of electrons around individual atoms. When atoms combine to form molecules, their atomic orbitals overlap, leading to the formation of molecular orbitals.
- Molecular Orbitals
Molecular orbitals are the wave functions that describe the behavior of electrons within a molecule. They are formed by the linear combination of atomic orbitals and can be classified as bonding or antibonding orbitals.
- Bonding Orbitals
Bonding molecular orbitals result from the constructive overlap of atomic orbitals and lead to a decrease in the energy of the system. Electrons occupying bonding orbitals contribute to the formation of chemical bonds.
- Antibonding Orbitals
Antibonding molecular orbitals result from the destructive overlap of atomic orbitals and lead to an increase in the energy of the system. Electrons occupying antibonding orbitals weaken or prevent the formation of chemical bonds.
MOT provides a powerful tool for calculating bond orders by analyzing the occupancy of molecular orbitals. The bond order is equal to the difference between the number of bonding and antibonding electrons. This approach is particularly useful for understanding the bonding in molecules with multiple bonds, such as carbon dioxide (CO2).
Hybridization
Hybridization is a fundamental concept in chemistry that describes the mixing of atomic orbitals to form new hybrid orbitals with different shapes and energies. It plays a crucial role in determining the bond order of carbon dioxide (CO2) and other molecules.
In the case of CO2, the carbon atom undergoes sp hybridization. This involves the mixing of one s orbital and two p orbitals to form two equivalent sp hybrid orbitals. These hybrid orbitals then overlap with the p orbitals of the two oxygen atoms, resulting in the formation of two sigma () bonds. The overlap of the remaining p orbitals on the carbon and oxygen atoms forms two pi () bonds.
The hybridization of the carbon atom in CO2 has a significant impact on its bond order. The sp hybrid orbitals have a more linear shape compared to pure p orbitals, which allows for better overlap with the p orbitals of the oxygen atoms. This results in the formation of stronger sigma bonds and, consequently, a higher bond order for CO2.
Understanding hybridization is essential for accurately calculating the bond order of CO2 and other molecules. It provides insights into the electronic structure and bonding characteristics of molecules, which is crucial for predicting their properties and reactivity. Applications of this understanding include the design of new materials, pharmaceuticals, and catalysts.
Resonance
Resonance is a key concept in chemistry that helps us understand the electronic structure and bonding of molecules, including carbon dioxide (CO2). It describes the delocalization of electrons across multiple equivalent positions or structures, providing a more accurate representation of the molecule’s electronic state.
- Delocalized Electrons
In resonance, electrons are not confined to a single bond or atom but are spread out across the molecule. This delocalization results in a more stable electronic configuration and lower energy state.
- Resonance Structures
Resonance structures are different Lewis structures that represent the same molecule. They have the same arrangement of atoms but differ in the placement of electrons. Resonance structures contribute to the overall resonance hybrid, which is a more accurate representation of the molecule’s electronic structure.
- Resonance Hybrid
The resonance hybrid is a weighted average of the resonance structures. It is a more stable and accurate representation of the molecule’s electronic structure than any single resonance structure.
Resonance plays a vital role in calculating the bond order of CO2. The two resonance structures of CO2 contribute to the overall resonance hybrid, which has a bond order of 1.5. This indicates that the carbon-oxygen bonds in CO2 have a double bond character, which is consistent with the molecule’s observed properties and reactivity.
Electronegativity
Electronegativity is a crucial concept in chemistry that measures the tendency of an atom to attract electrons towards itself. It plays a significant role in determining the bond order of carbon dioxide (CO2) and other molecules.
Electronegativity affects the distribution of electrons within a molecule, which in turn influences the bond order. A more electronegative atom will attract electrons more strongly, leading to a higher bond order. In the case of CO2, the oxygen atoms are more electronegative than the carbon atom. This means that the electrons in the CO2 molecule are drawn towards the oxygen atoms, resulting in a higher bond order between the carbon and oxygen atoms.
Understanding the relationship between electronegativity and bond order is essential for accurately calculating the bond order of CO2 and other molecules. It provides insights into the electronic structure and bonding characteristics of molecules, which is crucial for predicting their properties and reactivity. Applications of this understanding include the design of new materials, pharmaceuticals, and catalysts.
Bond Length
Bond length is a crucial aspect of understanding and calculating the bond order of carbon dioxide (CO2). It refers to the equilibrium distance between the nuclei of two bonded atoms and provides insights into the strength and nature of the chemical bond.
- Covalent Bond Length
In covalent bonds, the bond length is primarily determined by the atomic radii of the bonded atoms and the number of shared electron pairs. Shorter bond lengths indicate stronger bonds.
- Multiple Bonds
Multiple bonds, such as double or triple bonds, have shorter bond lengths compared to single bonds between the same atoms. This is because multiple bonds involve the sharing of more electron pairs, leading to increased electron density and stronger bonds.
- Electronegativity
Electronegativity, which measures the attraction of an atom for bonding electrons, affects bond length. Atoms with higher electronegativity tend to form shorter bonds.
- Hybridization
The hybridization of atomic orbitals also influences bond length. Orbitals with more s-character, such as sp or sp2 hybrid orbitals, result in shorter bond lengths.
Understanding bond length allows us to gain insights into the electronic structure and bonding characteristics of CO2 and other molecules. It aids in predicting molecular properties, such as bond strength, reactivity, and molecular geometry. Accurate determination of bond length is essential for various fields, including materials science, drug design, and catalysis.
Bond Energy
Bond energy is a crucial aspect of understanding and calculating the bond order of carbon dioxide (CO2). It refers to the energy required to break a chemical bond between two atoms and provides insights into the strength and nature of the bond.
The bond order of a molecule is directly related to its bond energy. A higher bond order typically corresponds to a stronger bond and, consequently, a higher bond energy. In the case of CO2, the bond order of 1.5 between the carbon and oxygen atoms indicates a relatively strong bond, which is reflected in the high bond energy of 805 kJ/mol required to break the C=O bond.
Understanding the relationship between bond energy and bond order allows us to gain insights into the stability and reactivity of molecules. Molecules with stronger bonds, indicated by higher bond energies and bond orders, are generally more stable and less reactive. Conversely, molecules with weaker bonds are more likely to undergo chemical reactions.
In practical applications, bond energy and bond order play a vital role in various fields. For example, in materials science, understanding bond energies helps design materials with specific properties, such as strength, durability, and thermal stability. In drug design, bond energies are considered to optimize the binding affinity of drugs to target molecules.
Magnetic Properties
Magnetic properties play a significant role in understanding and calculating the bond order of carbon dioxide (CO2). They provide insights into the electronic structure and bonding characteristics of CO2, complementing other methods used to determine bond order.
- Susceptibility
Magnetic susceptibility measures the degree to which a substance is magnetized when placed in a magnetic field. Diamagnetic substances have a negative susceptibility, while paramagnetic substances have a positive susceptibility. CO2 is a diamagnetic molecule, indicating that its electrons are paired and do not contribute to any net magnetic moment.
- Nuclear Magnetic Resonance (NMR)
NMR spectroscopy exploits the magnetic properties of atomic nuclei to provide detailed information about the structure and dynamics of molecules. Different atoms and isotopes have characteristic NMR frequencies, which can be used to identify and quantify atoms within a molecule. NMR has been extensively used to study the structure and bonding of CO2 and other molecules.
- Electron Paramagnetic Resonance (EPR)
EPR spectroscopy is a technique used to study paramagnetic species, which have unpaired electrons. EPR can provide information about the electronic structure of molecules and the nature of the paramagnetic center. While CO2 itself is diamagnetic, EPR has been applied to study paramagnetic species derived from CO2, such as the superoxide radical anion (O2-).
- Magnetic Moments
Magnetic moments arise from the motion of charged particles, including electrons. The magnetic moment of a molecule is determined by the sum of the magnetic moments of its constituent atoms and electrons. The magnetic moment of CO2 is zero due to the cancellation of the magnetic moments of the paired electrons.
Understanding the magnetic properties of CO2 provides valuable insights into its electronic structure and bonding. Magnetic susceptibility measurements confirm the diamagnetic nature of CO2, while NMR and EPR spectroscopy offer detailed information about the atomic and electronic structure. The combination of magnetic properties with other techniques enhances our understanding of the molecular properties and behavior of CO2.
Spectroscopic Properties
Spectroscopic properties play a crucial role in understanding and calculating the bond order of carbon dioxide (CO2). They provide valuable insights into the electronic structure and bonding characteristics of CO2, complementing other methods used to determine bond order.
- Infrared Spectroscopy
Infrared spectroscopy measures the absorption of infrared radiation by molecules. Functional groups with specific bond vibrations exhibit characteristic absorption frequencies. In the case of CO2, the asymmetric stretching vibration of the C=O bond appears at around 2350 cm-1, providing information about the bond strength and order.
- Raman Spectroscopy
Raman spectroscopy measures the inelastic scattering of light by molecules. It provides complementary information to infrared spectroscopy and can be used to probe vibrational modes that are not infrared-active. The Raman spectrum of CO2 exhibits a strong band at around 1388 cm-1, corresponding to the symmetric stretching vibration of the C=O bond.
- UV-Visible Spectroscopy
UV-visible spectroscopy measures the absorption of ultraviolet and visible radiation by molecules. Electronic transitions between molecular orbitals can be identified, providing insights into the electronic structure and bonding. CO2 exhibits weak absorption in the UV region, corresponding to transitions from the highest occupied molecular orbital (HOMO) to the lowest unoccupied molecular orbital (LUMO).
- Mass Spectrometry
Mass spectrometry measures the mass-to-charge ratio of ions. It can be used to identify and quantify different isotopes of CO2, providing information about the isotopic composition and abundance ratios. Mass spectrometry is also useful for studying fragmentation patterns and reaction pathways involving CO2.
By analyzing the spectroscopic properties of CO2, we gain insights into its molecular structure, vibrational modes, electronic transitions, and isotopic composition. This information contributes to a comprehensive understanding of the bond order and overall bonding characteristics of CO2.
FAQs on Bond Order Calculations in CO2
The following FAQs address common queries and provide further clarification on key aspects related to calculating the bond order of carbon dioxide (CO2):
Question 1: What is bond order and why is it important?
Answer: Bond order refers to the number of chemical bonds between two atoms. It is a crucial concept in chemistry as it provides insights into molecular stability, reactivity, and physical properties.
Question 2: How can we determine the bond order of CO2?
Answer: The bond order of CO2 can be calculated using various methods, including molecular orbital theory, hybridization, resonance, and electronegativity. Each method offers a unique perspective on the electronic structure and bonding characteristics of CO2.
Question 3: What role does hybridization play in bond order determination?
Answer: Hybridization involves the mixing of atomic orbitals to form new hybrid orbitals with different shapes and energies. In CO2, the carbon atom undergoes sp hybridization, which influences the bond order and geometry of the molecule.
Question 4: How does resonance affect the bond order of CO2?
Answer: Resonance is a phenomenon where electrons are delocalized across multiple equivalent positions or structures. In CO2, resonance contributes to the overall stability of the molecule and results in a bond order of 1.5, indicating a double bond character.
Question 5: What experimental techniques can provide insights into bond order?
Answer: Spectroscopic techniques such as infrared spectroscopy, Raman spectroscopy, and UV-visible spectroscopy can provide valuable information about the vibrational modes, electronic transitions, and isotopic composition of CO2, complementing theoretical calculations of bond order.
Question 6: How is bond order related to the properties of CO2?
Answer: Bond order is closely linked to the properties of CO2, such as its stability, reactivity, and physical characteristics. A higher bond order typically corresponds to a stronger and shorter bond, influencing the overall behavior and applications of CO2.
These FAQs provide a comprehensive overview of the key concepts and methods involved in calculating the bond order of CO2. Understanding bond order is essential for gaining insights into the electronic structure and properties of CO2, which has important implications in various fields of science and technology.
In the next section, we will delve deeper into the practical applications of bond order calculations in different areas, exploring how this concept contributes to our understanding and utilization of CO2.
Tips on Calculating Bond Order in CO2
The following tips provide practical guidance on accurately calculating the bond order of carbon dioxide (CO2):
Tip 1: Understand the Basic Concepts
Familiarize yourself with the fundamental concepts of bond order, molecular orbital theory, and hybridization.
Tip 2: Employ Various Methods
Utilize different methods such as the valence bond approach, molecular orbital theory, and resonance to cross-check your calculations.
Tip 3: Consider Hybridization
Determine the hybridization of the carbon atom in CO2 (sp) to understand the geometry and bonding characteristics.
Tip 4: Account for Resonance
Recognize that CO2 exhibits resonance, which contributes to the delocalization of electrons and affects the bond order.
Tip 5: Utilize Spectroscopic Data
Incorporate spectroscopic data, such as infrared and Raman spectroscopy, to validate your calculated bond order.
Tip 6: Leverage Computational Tools
Employ computational chemistry software to perform complex calculations and visualize molecular orbitals.
Tip 7: Understand the Implications
Interpret the calculated bond order in the context of CO2’s stability, reactivity, and physical properties.
Tip 8: Seek Expert Guidance
If needed, consult with experts in computational chemistry or quantum chemistry for assistance with advanced calculations.
By following these tips, you can enhance the accuracy and reliability of your bond order calculations for CO2. This understanding forms a solid foundation for further exploration of CO2’s properties and applications.
In the next section, we will delve into the significance of bond order calculations in various scientific disciplines, showcasing how this concept contributes to our understanding and utilization of CO2.
Conclusion
This in-depth exploration of bond order calculations in carbon dioxide (CO2) has provided valuable insights into the electronic structure and properties of this important molecule. Key takeaways include:
- Bond order calculations are crucial for understanding molecular stability, reactivity, and physical properties.
- Multiple methods, including molecular orbital theory, hybridization, and resonance, can be employed to calculate bond order accurately.
- The bond order of CO2 is 1.5, indicating a double bond character.
Understanding bond order is not only essential for CO2 chemistry but also has broader implications in various scientific disciplines. It enables researchers to design new materials, optimize chemical reactions, and develop technologies that harness CO2 for sustainable applications. As we delve further into the realm of molecular interactions, bond order calculations will continue to be a cornerstone of our understanding and utilization of chemical species.
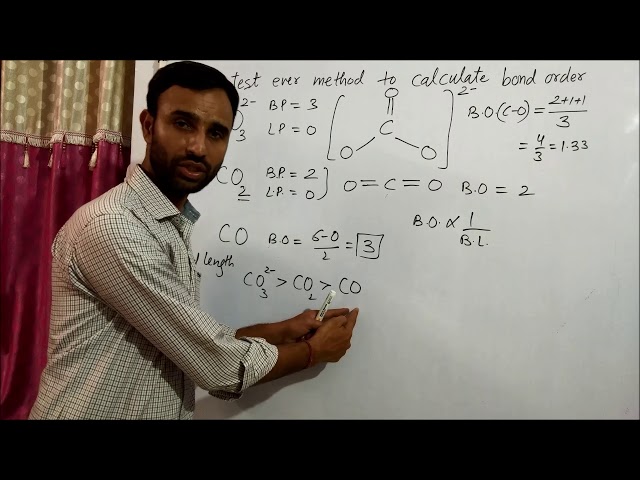