Bond order, a measure of the strength of a chemical bond, is a crucial concept in chemistry. Understanding how to calculate bond order of resonance structures is vital for comprehending molecular bonding and properties.
Resonance structures are representations of molecules that exhibit delocalization of electrons, resulting in multiple Lewis structures. Calculating bond order helps determine the relative strengths and lengths of bonds, providing insights into molecular stability and reactivity. The concept of resonance was first proposed by Linus Pauling in the 1930s, revolutionizing the understanding of chemical bonding.
This article will delve into the methods for calculating bond order of resonance structures, exploring the underlying principles and their applications in predicting molecular behavior.
How to Calculate Bond Order of Resonance Structures
Understanding how to calculate bond order of resonance structures is essential for comprehending molecular bonding and properties. Key aspects include:
- Resonance theory
- Delocalization
- Lewis structures
- Bond order
- Resonance structures
- Average bond order
- Quantum mechanics
- Molecular orbital theory
These aspects are interconnected and provide a deeper understanding of the behavior of molecules with delocalized electrons. Resonance theory explains the concept of resonance structures, which are different Lewis structures that represent the same molecule. Bond order, a measure of bond strength, can be calculated for each bond in a resonance structure. The average bond order, considering all resonance structures, provides insights into the overall bonding in the molecule. Quantum mechanics and molecular orbital theory offer a theoretical framework for understanding the delocalization of electrons and the formation of resonance structures.
Resonance Theory
Resonance theory is a fundamental concept in chemistry that explains the behavior of molecules with delocalized electrons. It provides a framework for understanding the bonding and properties of molecules that cannot be adequately described by a single Lewis structure. Resonance theory is critical for calculating bond order of resonance structures, as it allows us to consider the different contributing structures and their relative importance.
To calculate the bond order of a resonance structure, we need to determine the number of bonds between each pair of atoms. This is done by examining the Lewis structures of the resonance contributors and counting the number of bonds between each pair of atoms. The bond order is then calculated as the average number of bonds between each pair of atoms over all of the resonance contributors. For example, in the case of benzene, there are two resonance structures, each with six C-C bonds. The average bond order for each C-C bond is therefore 1.5.
Understanding resonance theory and how to calculate bond order of resonance structures is essential for comprehending the behavior of molecules with delocalized electrons. This knowledge has practical applications in various fields, including organic chemistry, inorganic chemistry, and biochemistry. For example, resonance theory is used to explain the stability of aromatic compounds, the reactivity of organic molecules, and the structure and function of biological molecules.
Delocalization
Delocalization, a central aspect of resonance theory, plays a crucial role in understanding how to calculate bond order of resonance structures. It describes the spreading out or delocalization of electrons over several atoms, resulting in a resonance hybrid that is more stable than any of the individual resonance structures.
- Electron Delocalization: Delocalization involves the delocalization of electrons, leading to a decrease in potential energy and increased stability of the molecule.
- Resonance Hybrid: Delocalized electrons create a resonance hybrid, which is a weighted average of the contributing resonance structures, representing the true electronic structure of the molecule.
- Bond Order: Delocalization affects bond order by distributing the electron density over multiple bonds, resulting in fractional bond orders and an overall decrease in bond strength.
- Implications: Delocalization has significant implications for chemical properties, such as stability, reactivity, and magnetic properties, and it plays a crucial role in various areas of chemistry, including organic chemistry and materials science.
Understanding delocalization is essential for accurately calculating bond order of resonance structures and gaining insights into the electronic structure and properties of molecules.
Lewis Structures
Lewis structures, a fundamental tool in chemistry, provide a simplified representation of the valence electrons in a molecule, atom, or ion. They are crucial for understanding how to calculate bond order of resonance structures, as they provide a visual representation of the electron distribution and bonding within the molecule.
- Skeletal Structure: A Lewis structure depicts the connectivity of atoms in a molecule, showing which atoms are bonded to each other.
- Electron Pairs: It represents valence electrons as dots or lines, indicating lone pairs and bonding pairs.
- Formal Charges: Lewis structures can be used to calculate formal charges on atoms, which can help determine the stability and reactivity of the molecule.
- Resonance Structures: Lewis structures are essential for drawing resonance structures, which represent the delocalization of electrons and are crucial for calculating bond order.
By understanding the components and implications of Lewis structures, we can accurately calculate bond order of resonance structures, gaining valuable insights into the electronic structure and properties of molecules.
Bond Order
Bond order is a crucial concept in chemistry, providing insights into the strength and nature of chemical bonds, especially when calculating bond order of resonance structures. It encompasses various aspects, each contributing to our understanding of molecular bonding and behavior:
- Bond Strength: Bond order directly correlates with bond strength, with higher bond orders indicating stronger bonds and shorter bond lengths.
- Electron Density: Bond order reflects the electron density between bonded atoms, with higher bond orders indicating greater electron density and stronger electrostatic attraction.
- Resonance Structures: In resonance structures, bond order helps determine the relative importance of each contributing structure and provides insights into the delocalization of electrons.
- Molecular Properties: Bond order influences molecular properties such as stability, reactivity, and magnetic behavior, aiding in predicting and understanding chemical reactions.
Understanding bond order, its components, and its implications is essential for accurately calculating bond order of resonance structures and gaining a deeper understanding of the electronic structure and properties of molecules.
Resonance structures
Resonance structures are a fundamental concept in chemistry, playing a pivotal role in understanding the electronic structure and bonding of molecules. They provide a means to represent the delocalization of electrons, which is crucial for accurately calculating bond order of resonance structures.
- Contributing Structures: Resonance structures are different Lewis structures that represent the same molecule. They arise when there is more than one possible arrangement of electrons that satisfies the octet rule for each atom.
- Delocalized Electrons: Resonance structures involve the delocalization of electrons, meaning that electrons are not confined to a specific bond or atom but are spread out over multiple atoms.
- Resonance Hybrid: The true electronic structure of a molecule with resonance is a resonance hybrid, which is a weighted average of the contributing resonance structures.
- Implications for Bond Order: Resonance structures affect bond order by distributing the electron density over multiple bonds, resulting in fractional bond orders and an overall decrease in bond strength.
By understanding the concept of resonance structures and its implications for bond order, chemists can gain deeper insights into the electronic structure and properties of molecules, including their stability, reactivity, and magnetic behavior.
Average Bond Order
Average bond order, a key concept in chemistry, is closely intertwined with understanding how to calculate bond order of resonance structures. It provides a means to determine the overall bond strength and electron distribution within molecules with resonance.
Calculating bond order of resonance structures involves considering the contributing resonance structures and their relative importance. By averaging the bond orders of these structures, we obtain the average bond order, which represents the overall bond strength and electron delocalization in the molecule. This concept is crucial for accurately predicting the stability, reactivity, and other properties of molecules with resonance.
Real-life examples of average bond order within the context of resonance structures include benzene and ozone. In benzene, the average bond order of the C-C bonds is 1.5, reflecting the delocalization of electrons around the ring. In ozone, the average bond order of the O-O bonds is 1.33, indicating resonance between two contributing structures.
Understanding average bond order has practical applications in various fields of chemistry. For instance, in organic chemistry, it helps predict the reactivity and stability of organic compounds. In inorganic chemistry, it aids in understanding the electronic structures and bonding of coordination complexes. Furthermore, average bond order is essential in computational chemistry for developing accurate models of molecular systems.
Quantum mechanics
Quantum mechanics plays a fundamental role in understanding how to calculate bond order of resonance structures. It provides the theoretical framework for describing the wave-particle duality of electrons and the delocalization of electrons in resonance structures.
- Wavefunction: The wavefunction describes the state of an electron and provides information about its probability of being found in a particular region of space. In resonance structures, the wavefunction is delocalized, indicating that the electrons are spread out over multiple atoms.
- Molecular orbitals: Molecular orbitals are mathematical functions that describe the wave-like behavior of electrons in molecules. In resonance structures, the molecular orbitals are delocalized, allowing electrons to move freely between different atoms.
- Resonance energy: Resonance energy is the difference in energy between the actual molecule and the hypothetical molecule with localized electrons. Resonance energy is a measure of the stability of the resonance hybrid and is related to the delocalization of electrons.
- Quantum superposition: Quantum superposition is the principle that an electron can exist in multiple states simultaneously. In resonance structures, the electrons are in a superposition of states, occupying multiple molecular orbitals at the same time.
By incorporating quantum mechanics into the calculation of bond order of resonance structures, we gain a deeper understanding of the electronic structure and bonding in molecules. This knowledge is essential for predicting the stability, reactivity, and other properties of molecules with resonance.
Molecular orbital theory
Molecular orbital theory (MOT) is a fundamental theory in quantum chemistry that describes the electronic structure of molecules. It provides a powerful framework for understanding the bonding and properties of molecules, including those with resonance structures. MOT is crucial for accurately calculating bond order of resonance structures, as it allows us to determine the molecular orbitals and their corresponding electron density distribution.
In MOT, electrons are treated as waves that occupy molecular orbitals, which are mathematical functions that describe the wave-like behavior of electrons within the molecule. The molecular orbitals are formed by the linear combination of atomic orbitals, and their shapes and energies depend on the symmetry and bonding interactions of the atoms in the molecule.
In the case of resonance structures, MOT provides a means to describe the delocalization of electrons over multiple atoms. Resonance structures arise when there is more than one possible arrangement of electrons that satisfies the octet rule for each atom. MOT allows us to calculate the molecular orbitals and electron density distribution for each resonance structure and then determine the overall bond order by averaging the bond orders of the individual resonance structures.
The application of MOT to resonance structures has significant implications for understanding the stability, reactivity, and other properties of molecules. For example, in benzene, MOT predicts that the six carbon atoms form a ring with delocalized electrons, resulting in resonance and an average bond order of 1.5 for each C-C bond. This delocalization of electrons contributes to the stability and unique properties of benzene.
Overall, MOT provides a rigorous and powerful framework for understanding how to calculate bond order of resonance structures. It allows us to determine the molecular orbitals and electron density distribution, which are essential for accurately predicting the properties and behavior of molecules with resonance.
FAQs on Calculating Bond Order of Resonance Structures
This section addresses frequently asked questions and clarifies key aspects of calculating bond order of resonance structures.
Question 1: What is resonance?
Answer: Resonance is a concept that describes the delocalization of electrons in a molecule, resulting in multiple Lewis structures that represent the same molecule.
Question 2: How do I calculate bond order for resonance structures?
Answer: To calculate bond order, you need to consider all resonance structures and determine the average number of bonds between each pair of atoms over all resonance structures.
Question 3: What is the significance of bond order in resonance structures?
Answer: Bond order provides insights into the strength and length of bonds, helping predict molecular stability and reactivity.
Question 4: How does resonance affect bond order?
Answer: Resonance delocalizes electrons, distributing them over multiple bonds. This leads to fractional bond orders and an overall decrease in bond strength.
Question 5: What is the relationship between resonance energy and bond order?
Answer: Resonance energy, which measures the stability of resonance structures, is directly related to bond order. Higher resonance energy corresponds to lower bond orders.
Question 6: How is bond order used in chemistry?
Answer: Bond order is a crucial concept in understanding molecular properties, predicting reactivity, and designing new materials.
In summary, calculating bond order of resonance structures involves understanding resonance, considering all resonance structures, and applying appropriate mathematical techniques. It provides valuable insights into molecular bonding and properties.
Delving deeper into the topic, the next section will explore advanced concepts and applications of bond order calculations in resonance structures.
Tips for Calculating Bond Order of Resonance Structures
This section presents practical tips to enhance your understanding and accuracy when calculating bond order of resonance structures.
Tip 1: Identify all possible resonance structures to ensure a comprehensive analysis.
Tip 2: Draw resonance structures that obey the octet rule for each atom involved.
Tip 3: Determine the bond order for each resonance structure by counting the number of bonds between each pair of atoms.
Tip 4: Calculate the average bond order by summing the bond orders of all resonance structures and dividing by the number of resonance structures.
Tip 5: Use molecular orbital theory to gain deeper insights into the delocalization of electrons and its impact on bond order.
Tip 6: Consider the symmetry of the molecule to simplify the identification of resonance structures.
Tip 7: Utilize computational tools or software to assist with complex resonance structures.
Tip 8: Validate your results by comparing them with experimental data or other theoretical calculations.
By following these tips, you can effectively calculate bond order of resonance structures, gaining valuable insights into molecular bonding and properties.
As we conclude the discussion on bond order calculations, the next section will delve into the applications of this concept in understanding molecular stability, reactivity, and other chemical phenomena.
Conclusion
This article has explored the intricacies of calculating bond order of resonance structures, a fundamental concept in understanding the electronic structure and bonding of molecules. By examining resonance theory, considering all resonance structures, and applying appropriate mathematical techniques, we gain valuable insights into molecular properties and behavior.
Key points to remember include:
- Resonance structures arise from the delocalization of electrons, leading to multiple Lewis structures for the same molecule.
- Bond order in resonance structures is a measure of bond strength and is calculated as the average number of bonds between each pair of atoms over all resonance structures.
- Bond order is crucial for predicting molecular stability, reactivity, and other chemical phenomena.
Understanding bond order of resonance structures is not merely an academic exercise but empowers chemists to design new materials, predict reaction outcomes, and unravel the complexities of the molecular world.
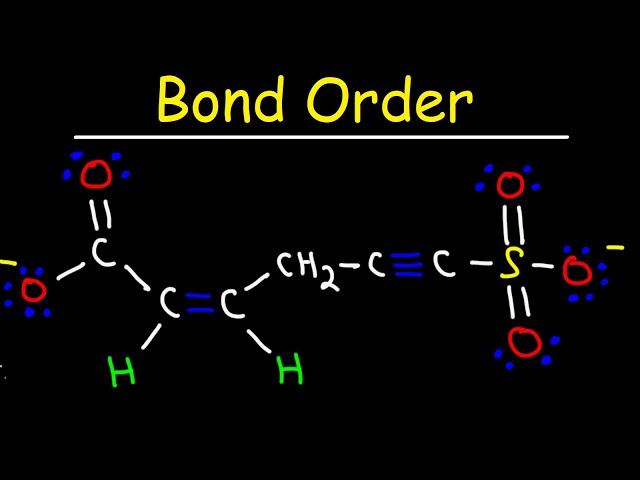