Bond order is a chemical concept that describes the number of chemical bonds between atoms in a molecule. It is a crucial factor in determining the stability, reactivity, and properties of a molecule. To effectively grasp the notion of bond order, understanding how to calculate it is paramount.
In this article, we will delve into the calculation of bond order, using cyanide as an example. Cyanide is a highly reactive compound that finds applications in various industries, including electroplating, metallurgy, and the production of plastics. Understanding the bond order of cyanide is essential for comprehending its chemical behavior and devising strategies for its safe and effective utilization.
Our exploration will encompass the fundamental principles governing bond order calculations, examining the underlying theories and methodologies. We will also highlight the significance of bond order in understanding molecular structure, reactivity, and bonding.
How to Calculate Bond Order of Cyanide
Calculating bond order is crucial for understanding the chemical behavior of cyanide. Key aspects to consider include:
- Molecular orbital theory
- Electron configuration
- Hybridization
- Resonance
- Delocalization
- Electronegativity
- Bond length
- Bond strength
- Magnetic properties
- Spectroscopic data
These aspects are interconnected and provide valuable insights into the nature of the bond between carbon and nitrogen in cyanide. Understanding bond order allows chemists to predict and explain the reactivity, stability, and properties of cyanide, enabling its safe and effective use in various applications.
Molecular orbital theory
Molecular orbital theory (MOT) is a fundamental concept in quantum chemistry used to describe the electronic structure of molecules. It explains the bonding and properties of molecules based on the behavior of electrons in molecular orbitals, which are mathematical functions describing the wave-like behavior of electrons in the molecule.
In the context of calculating bond order, MOT is critical because it provides a framework for understanding the electronic structure of cyanide. By applying MOT, chemists can determine the number and type of molecular orbitals formed from the atomic orbitals of carbon and nitrogen, and how these orbitals interact to form the chemical bond.
A real-life example of the application of MOT in calculating bond order is the use of Hckel molecular orbital theory to determine the bond order of benzene. Hckel theory, a simplified version of MOT, allows for the calculation of bond orders in conjugated systems, such as benzene, and has been successfully used to explain the stability and reactivity of these systems.
Understanding the connection between MOT and bond order calculations has practical applications in various fields, including inorganic chemistry, organic chemistry, and materials science. By accurately calculating bond order, chemists can predict and explain the chemical behavior of molecules, design new materials with tailored properties, and develop new synthetic methods for the production of useful compounds.
Electron configuration
Electron configuration, the distribution of electrons in atomic orbitals, plays a pivotal role in determining the bond order of cyanide. Cyanide, with its unique electronic structure, provides an ideal case study to explore this connection. The number and arrangement of electrons in the atomic orbitals of carbon and nitrogen govern the formation and properties of the cyanide bond.
To calculate the bond order of cyanide, we examine the molecular orbitals formed by the overlap of atomic orbitals. The electron configuration of carbon (1s2 2s2 2p2) and nitrogen (1s2 2s2 2p3) suggests that the 2p orbitals of both atoms participate in the bonding. The overlap of these orbitals results in the formation of two molecular orbitals: a bonding orbital and an antibonding * orbital.
The bond order is directly related to the number of electrons occupying the bonding and antibonding orbitals. In cyanide, both the bonding and antibonding orbitals are filled with two electrons, resulting in a bond order of one. This indicates a single bond between carbon and nitrogen, consistent with the Lewis structure of cyanide (CN). Understanding the electron configuration and its impact on bond order allows chemists to accurately predict the molecular structure and properties of cyanide.
Hybridization
Hybridization refers to the phenomenon where atomic orbitals combine to form new hybrid orbitals with different shapes and energies. In the context of cyanide, hybridization plays a critical role in determining the bond order and molecular geometry.
The carbon atom in cyanide undergoes sp hybridization, which involves the mixing of one 2s orbital and one 2p orbital to form two sp hybrid orbitals. These sp hybrid orbitals then overlap with the 2p orbitals of nitrogen to form the CN bond. The triple bond in cyanide consists of one bond and two bonds, resulting in a bond order of three.
Understanding hybridization is essential for accurately calculating the bond order of cyanide. Without considering hybridization, the bond order would be incorrectly determined as one, based solely on the number of electron pairs shared between carbon and nitrogen. Real-life applications of this understanding include the design of new materials with tailored properties and the development of new synthetic methods for the production of useful compounds.
Resonance
Resonance is a fundamental concept in chemistry that describes the delocalization of electrons within a molecule or ion. It occurs when multiple Lewis structures can be drawn for a molecule, and no single Lewis structure can fully represent the molecule’s electronic structure. Resonance is particularly important in understanding the bonding in cyanide, as it contributes to the stability and unique properties of the cyanide ion.
In the case of cyanide, resonance occurs due to the presence of a triple bond between carbon and nitrogen. The carbon atom in cyanide is sp hybridized, and its two sp hybrid orbitals overlap with two of the nitrogen atom’s p orbitals to form two bonds. The third bond between carbon and nitrogen is formed by the overlap of the remaining p orbitals on both atoms, resulting in a bond. However, the bond in cyanide is not localized between a specific pair of atoms; instead, it is delocalized over the entire molecule. This delocalization is represented by the two resonance structures of cyanide:
NC- :: CN-
The concept of resonance is critical in calculating the bond order of cyanide. Without considering resonance, the bond order of cyanide would be incorrectly determined as one, based solely on the number of electron pairs shared between carbon and nitrogen. However, the resonance structures show that the bond order in cyanide is actually three, indicating the presence of a triple bond.
Understanding resonance and its impact on bond order has practical applications in various fields of chemistry. For example, in inorganic chemistry, resonance is used to explain the stability of complex ions, such as the hexacyanoferrate(II) ion ([Fe(CN)6]4-). In organic chemistry, resonance is used to explain the reactivity and regioselectivity of organic reactions. Additionally, resonance is important in understanding the electronic structure of materials, such as semiconductors and superconductors.
Delocalization
Delocalization, a crucial concept in chemistry, plays a significant role in understanding how to calculate the bond order of cyanide. It pertains to the dispersal of electrons over multiple atoms or regions within a molecule, defying the notion of localized bonds between specific atom pairs. Delocalization has profound implications for the electronic structure, stability, and reactivity of cyanide, offering a more nuanced perspective beyond traditional bonding models.
- Resonance Structures
Resonance structures are alternative representations of a molecule that illustrate the delocalization of electrons. In cyanide, resonance structures show the triple bond between carbon and nitrogen as delocalized over the entire molecule, contributing to its stability and unique properties.
- Molecular Orbitals
Molecular orbital theory describes the behavior of electrons in molecules using wave functions. Delocalized electrons occupy molecular orbitals that extend over multiple atoms, leading to the formation of bonds in cyanide.
- Aromaticity
Aromaticity, a special type of delocalization, occurs when electrons are cyclically delocalized over a planar ring structure. Cyanide does not exhibit aromaticity, but understanding the concept provides insights into related aromatic compounds.
- Implications for Bond Order
Delocalization affects bond order calculations by distributing the bond order over multiple bonds. In cyanide, the triple bond is not localized between a specific carbon-nitrogen pair, but rather delocalized over the entire molecule, resulting in a bond order of three.
Delocalization offers a deeper understanding of the electronic structure of cyanide, enabling more accurate bond order calculations and predictions of its chemical behavior. This concept is not limited to cyanide but extends to various molecules and materials, highlighting its significance in diverse areas of chemistry.
Electronegativity
Electronegativity, a fundamental property of atoms, plays a crucial role in determining the bond order of cyanide. It measures the tendency of an atom to attract electrons towards itself, influencing the distribution of electron density within the molecule.
- Pauling Electronegativity
Quantifies the electronegativity of atoms on a scale from 0 to 4, with higher values indicating a greater attraction for electrons. Carbon and nitrogen, the constituent atoms of cyanide, have Pauling electronegativities of 2.5 and 3.0, respectively.
- Electronegativity Difference
The difference in electronegativity between bonded atoms affects the polarity of the bond. In cyanide, the electronegativity difference between carbon and nitrogen is 0.5, resulting in a slightly polar bond with a partial negative charge on nitrogen.
- Electronegativity and Bond Order
Electronegativity influences bond order by affecting the distribution of electron density in the molecular orbitals. In cyanide, the higher electronegativity of nitrogen draws electron density towards itself, strengthening the bond and increasing the bond order.
- Electronegativity and Resonance
Electronegativity also influences resonance by affecting the stability of resonance structures. In cyanide, the electronegative nitrogen atom stabilizes the resonance structure where it bears a negative charge, contributing to the overall stability of the molecule.
Understanding electronegativity and its multifaceted aspects is essential for accurately calculating the bond order of cyanide and predicting its chemical behavior. The interplay between electronegativity, bond polarity, resonance, and bond order provides valuable insights into the electronic structure and properties of this important molecule.
Bond length
Bond length, a crucial aspect of molecular structure, plays a significant role in determining the bond order of cyanide. It refers to the distance between the nuclei of two bonded atoms and provides valuable insights into the nature of the chemical bond.
The bond length in cyanide is inversely related to the bond order. A shorter bond length typically indicates a stronger bond and higher bond order. In cyanide, the carbon-nitrogen bond has a bond length of about 1.16 angstroms, which corresponds to a bond order of three. This suggests the presence of a strong triple bond between carbon and nitrogen.
Understanding the relationship between bond length and bond order is essential for accurately calculating the bond order of cyanide. Various experimental techniques, such as X-ray crystallography and microwave spectroscopy, can be used to determine the bond length, which then aids in calculating the bond order.
In practical applications, the bond length and bond order of cyanide are important factors in understanding its reactivity and stability. For example, the short bond length and high bond order of the carbon-nitrogen triple bond contribute to cyanide’s toxicity and its use as a ligand in coordination complexes.
Bond strength
Bond strength is a crucial aspect of understanding the chemical behavior of cyanide and plays a significant role in determining its bond order. It refers to the strength of the attractive force between two bonded atoms, influencing the stability and reactivity of the molecule.
- Bond Dissociation Energy
Bond dissociation energy measures the energy required to break a bond between two atoms. In cyanide, the carbon-nitrogen bond has a high bond dissociation energy, indicating a strong bond.
- Bond Length
Bond length, the distance between the nuclei of two bonded atoms, is inversely related to bond strength. The shorter the bond length, the stronger the bond. Cyanide has a relatively short carbon-nitrogen bond length, contributing to its high bond strength.
- Electronegativity
Electronegativity, the ability of an atom to attract electrons, affects bond strength. The difference in electronegativity between carbon and nitrogen leads to a polar bond in cyanide, contributing to its overall bond strength.
- Resonance
Resonance, the delocalization of electrons over multiple atoms, can enhance bond strength. In cyanide, resonance structures contribute to the stability of the molecule, increasing the overall bond strength.
Understanding bond strength and its various components is essential for accurately calculating the bond order of cyanide. These factors provide insights into the nature of the carbon-nitrogen bond and its influence on the molecule’s chemical properties.
Magnetic properties
Magnetic properties play a vital role in understanding the electronic structure and bonding in cyanide. Paramagnetism, a type of magnetism that arises from the presence of unpaired electrons, is a crucial property to consider when calculating the bond order of cyanide.
The presence of unpaired electrons in a molecule can affect the bond order. In cyanide, the carbon atom has two unpaired electrons, while the nitrogen atom has three. The interaction between these unpaired electrons can lead to a decrease in the bond order. Paramagnetism is directly related to the number of unpaired electrons, and by measuring the magnetic properties of cyanide, we can gain insights into the bond order.
Real-life applications of this understanding include the use of magnetic susceptibility measurements to determine the bond order of cyanide in various compounds. This information is valuable in understanding the electronic structure and reactivity of cyanide-containing molecules. Additionally, the magnetic properties of cyanide are important in the field of coordination chemistry, where cyanide is often used as a ligand to form complexes with transition metal ions.
Spectroscopic data
Spectroscopic data plays a crucial role in understanding the electronic structure and bonding in cyanide, providing valuable insights for calculating its bond order. Various spectroscopic techniques offer complementary information that aids in determining the bond order.
- UV-Vis spectroscopy measures the absorption of ultraviolet and visible light by molecules. In cyanide, UV-Vis spectroscopy can provide information about the electronic transitions within the molecule, which can be related to the bond order.
- Infrared spectroscopy measures the absorption of infrared radiation by molecules. In cyanide, infrared spectroscopy can provide information about the vibrational modes of the molecule, which can be used to determine the bond strengths and bond order.
- NMR spectroscopy measures the magnetic properties of atomic nuclei. In cyanide, NMR spectroscopy can provide information about the electronic environment of the carbon and nitrogen atoms, which can be used to determine the bond order.
- Electron paramagnetic resonance (EPR) spectroscopy measures the absorption of microwave radiation by molecules with unpaired electrons. In cyanide, EPR spectroscopy can provide information about the number of unpaired electrons in the molecule, which can be used to determine the bond order.
These spectroscopic techniques, when combined with other experimental data and theoretical calculations, provide a comprehensive understanding of the electronic structure and bonding in cyanide, enabling accurate determination of its bond order. Spectroscopic data is not only essential for understanding cyanide but also for studying various chemical systems, providing insights into their structure, bonding, and properties.
Frequently Asked Questions
This section addresses common questions and clarifies aspects related to calculating the bond order of cyanide.
Question 1: What is bond order and why is it important in understanding cyanide?
Answer: Bond order describes the number of chemical bonds between atoms in a molecule. For cyanide, understanding bond order is crucial for comprehending its stability, reactivity, and applications.
Question 2: How can I calculate the bond order of cyanide?
Answer: Calculating bond order involves considering molecular orbital theory, electron configuration, hybridization, resonance, delocalization, electronegativity, bond length, bond strength, magnetic properties, and spectroscopic data.
Question 3: What is the relationship between electronegativity and bond order in cyanide?
Answer: The difference in electronegativity between carbon and nitrogen polarizes the bond, influencing the distribution of electron density and affecting the bond order.
Question 4: How does resonance affect the bond order of cyanide?
Answer: Resonance stabilizes the cyanide ion by delocalizing the bond over the entire molecule, contributing to the observed bond order.
Question 5: Can spectroscopic techniques provide insights into the bond order of cyanide?
Answer: Yes, UV-Vis, infrared, NMR, and EPR spectroscopy offer complementary information about the electronic structure and bonding in cyanide, aiding in the determination of bond order.
Question 6: What are some practical applications of understanding bond order in cyanide?
Answer: Understanding bond order helps predict and explain the reactivity, stability, and properties of cyanide, enabling its safe and effective use in various industrial and research applications.
These FAQs provide a concise overview of the key concepts and considerations involved in calculating the bond order of cyanide. Delving deeper into these aspects will further enhance our understanding of the electronic structure and chemical behavior of cyanide.
Next, we will explore the advanced applications of bond order calculations, discussing how this knowledge contributes to the design of new materials, the development of novel synthetic methods, and the elucidation of complex chemical reactions.
Tips for Calculating Bond Order of Cyanide
This section provides practical tips to assist in accurately calculating the bond order of cyanide. By following these tips, researchers and students can enhance their understanding of cyanide’s electronic structure and chemical behavior.
Tip 1: Understand Molecular Orbital Theory
Comprehending the principles of molecular orbital theory lays the foundation for understanding bond order calculations.
Tip 2: Determine Electron Configuration
Accurately determining the electron configuration of carbon and nitrogen is essential for calculating bond order.
Tip 3: Consider Hybridization
Hybridization of atomic orbitals significantly influences bond order, and it must be taken into account.
Tip 4: Analyze Resonance Structures
Resonance can affect bond order by delocalizing electrons. Identifying resonance structures is crucial.
Tip 5: Utilize Delocalization Concept
Delocalization of electrons can impact bond order. Understanding this concept provides a more accurate representation of bonding.
Tip 6: Account for Electronegativity
Electronegativity influences electron distribution and bond order. Consider the electronegativity difference between carbon and nitrogen.
Tip 7: Measure Bond Length
Bond length is inversely related to bond order. Experimental techniques can provide valuable bond length data.
Tip 8: Examine Magnetic Properties
Unpaired electrons can affect bond order. Magnetic susceptibility measurements can reveal insights into the number of unpaired electrons.
By incorporating these tips into bond order calculations, researchers can obtain more precise and reliable results. This knowledge deepens our understanding of cyanide’s electronic structure and aids in predicting its chemical behavior.
The subsequent section will delve into advanced applications of bond order calculations, demonstrating how this concept empowers chemists to design new materials, develop synthetic methods, and elucidate complex reactions.
Conclusion
This comprehensive exploration of bond order calculations in cyanide has illuminated the intricate relationship between molecular structure and chemical bonding. Key findings include the significance of molecular orbital theory, hybridization, resonance, and electronegativity in determining bond order. Understanding these concepts provides a deeper insight into cyanide’s stability, reactivity, and diverse applications.
The interplay between bond order and properties such as bond length, bond strength, and magnetic susceptibility further underscores the importance of accurate bond order calculations. Spectroscopic techniques, such as UV-Vis, infrared, NMR, and EPR spectroscopy, offer valuable experimental data to complement theoretical calculations and validate bond order determinations.
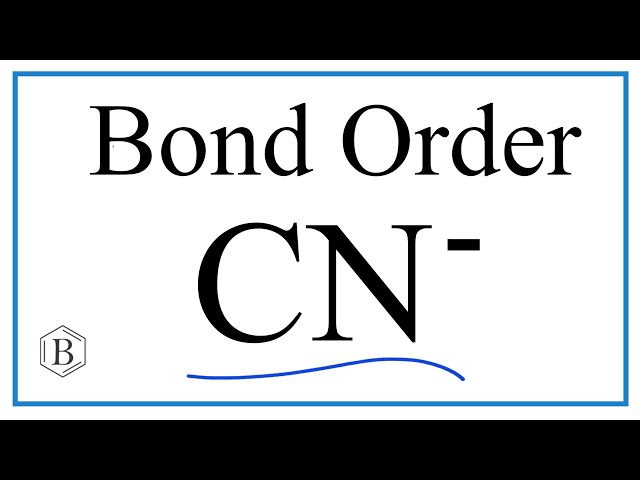