Calculating bond order for carbon monoxide (CO) is a cornerstone of chemistry, enabling us to probe the nature of its chemical bond. CO’s unique properties, due in part to its bond characteristics, make it indispensable in a myriad of industrial processes, including the production of fuels, plastics, and pharmaceuticals.
Understanding how to calculate bond order for CO opens doors to comprehending its reactivity and properties. Historically, the concept of bond order emerged from the pioneering work of Linus Pauling in the 1930s, providing a powerful tool to describe the strength and nature of chemical bonds.
In this article, we will delve into the intricacies of calculating bond order for CO, exploring the underlying principles and practical applications of this fundamental concept in chemistry.
how to calculate bond order for co
Determining the bond order of carbon monoxide (CO) is a fundamental aspect of understanding its chemical properties and behavior. Key factors involved in this calculation include:
- Atomic orbitals
- Electron configuration
- Molecular orbital theory
- Bond length
- Bond strength
- Molecular symmetry
- Resonance
- Hybridization
These aspects are interconnected and provide a comprehensive understanding of the electronic structure and bonding in CO. By considering these factors, chemists can accurately calculate the bond order, which is a valuable parameter in predicting and explaining the molecule’s properties and reactivity.
Atomic orbitals
When delving into “how to calculate bond order for CO,” comprehending atomic orbitals is paramount. They are the foundation upon which molecular orbitals are built, which in turn dictate the chemical bonding and properties of CO. Atomic orbitals describe the three-dimensional space where electrons are most likely to be found around an atom’s nucleus.
In the context of CO, the atomic orbitals of carbon and oxygen atoms overlap to form molecular orbitals. The number and type of these molecular orbitals determine the bond order of CO. For instance, the overlap of the carbon 2p atomic orbital with the oxygen 2p atomic orbital results in the formation of a sigma molecular orbital, contributing to the overall bond order of CO.
Understanding atomic orbitals empowers chemists to determine the electronic structure and bonding characteristics of CO, enabling predictions about its reactivity and behavior. Practical applications include the design of catalysts tailored for specific chemical reactions, the development of new materials with enhanced properties, and the optimization of industrial processes involving CO.
Electron configuration
Determining the electron configuration of carbon monoxide (CO) is a crucial aspect of calculating its bond order. Electron configuration describes the distribution of electrons in atomic orbitals, providing insights into the chemical bonding and properties of CO.
- Atomic Orbitals: Atomic orbitals are the three-dimensional regions around the nucleus where electrons are most likely to be found.
- aufbau principle: Electrons occupy the lowest energy orbitals available, following specific rules.
- Hund’s Rule: Electrons in the same orbital have parallel spins, maximizing the total spin of the atom.
- Pauli Exclusion Principle: No two electrons can have the same set of quantum numbers.
Understanding electron configuration enables chemists to determine the number and type of molecular orbitals formed in CO, which in turn dictate the bond order. This understanding is essential for predicting and explaining the reactivity and behavior of CO in various chemical processes, including its role in combustion, catalysis, and the production of fuels and pharmaceuticals.
Molecular orbital theory
Molecular orbital theory (MOT) is a cornerstone of quantum chemistry, offering a powerful framework to understand the electronic structure and bonding in molecules. It is the lynchpin connecting “how to calculate bond order for CO” and the fundamental principles governing chemical bonding.
MOT posits that the electrons in a molecule occupy molecular orbitals, which are formed by the mathematical combination of atomic orbitals. These molecular orbitals are characterized by their energy, shape, and symmetry. The number and type of molecular orbitals formed dictate the overall electronic structure and properties of the molecule.
In the case of CO, MOT enables the calculation of bond order by determining the number of electrons occupying the bonding and antibonding molecular orbitals. The bond order is directly proportional to the number of bonding electrons and inversely proportional to the number of antibonding electrons. This understanding allows chemists to predict the strength and nature of the chemical bond in CO, which is crucial for comprehending its reactivity and behavior.
Practical applications of molecular orbital theory in calculating bond order for CO abound. For instance, it guides the design of catalysts tailored for specific chemical reactions, where the bond order between the catalyst and reactants/products influences the catalytic efficiency. It also aids in the development of new materials with enhanced properties, as the bond order within the material determines its strength, stability, and electronic characteristics.
In summary, molecular orbital theory provides the theoretical underpinnings for calculating bond order for CO, enabling chemists to understand and predict the electronic structure, bonding, and properties of this important molecule. This understanding serves as a foundation for a wide range of applications in chemistry, materials science, and beyond.
Bond length
Bond length, a fundamental property of molecules, plays a pivotal role in determining the bond order of carbon monoxide (CO). It provides insights into the strength and nature of the chemical bond, offering valuable information for understanding CO’s reactivity and behavior.
- Interatomic Distance: The distance between the nuclei of the bonded carbon and oxygen atoms, a direct measure of bond length, influences the overlap of atomic orbitals and the strength of the bond.
- Bond Strength: Bond length and bond strength are inversely related. Shorter bond lengths indicate stronger bonds due to increased overlap of atomic orbitals, leading to greater electron density and attraction between the nuclei.
- Resonance Structures: In CO, resonance structures involving double and triple bonds contribute to the overall bond order and bond length. The presence of resonance structures can affect the observed bond length compared to a simple Lewis structure.
- Hybridization: The hybridization of carbon and oxygen atomic orbitals influences the bond length. sp-hybridized carbon and oxygen orbitals result in a shorter bond length and stronger bond compared to sp2– or sp3-hybridized orbitals.
Understanding bond length is crucial for accurately calculating the bond order of CO, which in turn provides insights into its electronic structure, reactivity, and properties. This knowledge is essential in diverse fields such as catalysis, materials science, and atmospheric chemistry, where CO plays a significant role.
Bond strength
Bond strength, an intrinsic property of chemical bonds, holds immense significance in understanding “how to calculate bond order for co.” It reflects the strength of the attractive forces between atoms, impacting the stability, reactivity, and behavior of molecules.
- Bond Dissociation Energy: The energy required to break a bond, providing a direct measure of bond strength. It is crucial for calculating bond order as it reflects the stability of the bond.
- Bond Length: Bond strength and bond length are inversely related. Shorter bond lengths indicate stronger bonds due to increased overlap of atomic orbitals. This relationship is considered when determining bond order.
- Resonance: Resonance structures, involving the delocalization of electrons, can influence bond strength. Resonance structures contribute to the overall bond order, affecting the strength of individual bonds.
- Hybridization: The hybridization of atomic orbitals affects bond strength. Stronger bonds are formed when orbitals with greater overlap are involved. This hybridization must be taken into account for accurate bond order calculations.
Understanding bond strength is essential for calculating bond order in CO, enabling the prediction of its chemical properties and reactivity. This knowledge finds applications in diverse fields, including the design of catalysts, the development of new materials, and the understanding of atmospheric chemistry.
Molecular symmetry
Molecular symmetry plays a significant role in determining the bond order of carbon monoxide (CO). It provides insights into the arrangement of atoms and the distribution of electrons within the molecule, influencing its overall properties and behavior.
- Point group symmetry: CO belongs to the Cv point group, indicating its cylindrical symmetry. This symmetry constrains the possible molecular orbitals and their interactions, affecting the bond order.
- Linear geometry: CO’s linear geometry results from the sp hybridization of the carbon atom and the p atomic orbital of the oxygen atom. This linear arrangement optimizes orbital overlap and contributes to the strong bond order in CO.
- Dipole moment: CO possesses a permanent dipole moment due to the electronegativity difference between carbon and oxygen. The dipole moment influences the interactions of CO with other molecules and surfaces, impacting its reactivity.
- Sigma and pi bonds: CO’s bond order arises from the combination of a sigma bond (formed by the overlap of sp orbitals) and a pi bond (formed by the overlap of p orbitals). The symmetry properties of these orbitals dictate their interactions and contributions to the overall bond order.
Understanding molecular symmetry is crucial for accurately calculating the bond order of CO, enabling predictions about its electronic structure, spectroscopic properties, and chemical reactivity. This knowledge finds applications in diverse fields such as catalysis, materials science, and atmospheric chemistry, where CO plays a vital role.
Resonance
Resonance is a crucial concept in chemistry that deeply influences “how to calculate bond order for CO.” It arises from the phenomenon of delocalization, where electrons are not confined to a specific pair of atoms but are spread across multiple atomic centers. This delocalization affects the overall electronic structure and bonding in CO, impacting its bond order calculation.
In CO, resonance occurs due to the presence of multiple Lewis structures with different bond arrangements. Each resonance structure contributes to the overall electronic structure, and the true bonding situation is a hybrid of these structures. This resonance affects the bond order calculation as it influences the number of bonding and antibonding electrons, which directly determine the bond order.
For instance, in CO, the resonance between a double bond and a triple bond contributes to the bond order. The double bond structure has two bonding electrons, while the triple bond structure has three bonding electrons. The actual bond order in CO is an average of these two contributions, resulting in a bond order of 2.5. This resonance-based understanding of bond order helps explain CO’s unique properties and reactivity.
Comprehending the connection between resonance and bond order calculation for CO has practical applications in diverse fields. It aids in designing catalysts with tailored activity and selectivity, as the bond order influences the interaction between the catalyst and reactants. Furthermore, it is essential in understanding CO’s role in atmospheric chemistry and its impact on environmental processes.
Hybridization
Hybridization is a fundamental concept in chemistry that provides a deeper understanding of the electronic structure and bonding in molecules. It plays a critical role in determining the bond order of carbon monoxide (CO), influencing its properties and reactivity.
Hybridization involves the mixing of atomic orbitals to form new hybrid orbitals with different shapes and energies. In the case of CO, the carbon atom undergoes sp hybridization, where one 2s atomic orbital and one 2p atomic orbital combine to form two sp hybrid orbitals. These sp hybrid orbitals then overlap with the 2p atomic orbital of the oxygen atom, resulting in the formation of a sigma bond and a pi bond.
The hybridization of the carbon atom affects the bond order of CO. The sp hybridization leads to a stronger sigma bond compared to a pure p-p bond, as the sp hybrid orbitals have a greater overlap with the oxygen 2p orbital. This stronger sigma bond contributes to a higher bond order for CO.
Understanding the connection between hybridization and bond order calculation for CO has practical applications in various fields. For instance, in catalysis, the hybridization of metal atoms in catalysts can influence the strength and selectivity of the catalyst for specific reactions. Additionally, in materials science, the hybridization of atoms in materials can affect their electronic properties, such as conductivity and optical properties.
Frequently Asked Questions
This section addresses common questions and clarifications regarding “how to calculate bond order for co”.
Question 1: What is bond order and why is it important for CO?
Answer: Bond order describes the strength and nature of the chemical bond between atoms. For CO, bond order is crucial for understanding its stability, reactivity, and properties.
Question 2: How do I calculate the bond order of CO?
Answer: Bond order for CO can be calculated using various methods, including the molecular orbital theory approach, which involves determining the number of bonding and antibonding electrons.
Question 3: What factors influence the bond order of CO?
Answer: Factors affecting CO’s bond order include atomic orbital overlap, electron configuration, hybridization, and resonance.
Question 4: How does bond order affect the properties of CO?
Answer: Bond order influences CO’s bond length, bond strength, and molecular stability, impacting its reactivity and behavior in chemical reactions.
Question 5: What are the applications of understanding bond order for CO?
Answer: Comprehending CO’s bond order has applications in catalysis, materials science, and atmospheric chemistry, aiding in the design of efficient catalysts, novel materials, and understanding environmental processes.
Question 6: Are there any limitations to calculating bond order for CO?
Answer: While methods for calculating bond order provide valuable insights, they may have limitations in certain situations, such as when dealing with complex molecular systems or highly accurate calculations.
These FAQs provide a concise overview of key aspects related to “how to calculate bond order for co”. Further discussion will delve into the practical applications and implications of bond order in understanding CO’s chemistry.
Transition to the next section: Understanding the bond order of CO is essential for exploring its reactivity and properties. In the next section, we will investigate the applications of bond order in various chemical contexts.
Tips for Calculating Bond Order for CO
In this section, we present practical tips and strategies to assist in accurately calculating the bond order of carbon monoxide (CO). By following these guidelines, you can enhance your understanding of CO’s electronic structure and bonding characteristics.
Tip 1: Determine the atomic orbital overlap between carbon (2p) and oxygen (2p) orbitals, as this overlap forms the basis for the sigma bond in CO.
Tip 2: Consider the hybridization of the carbon atom, typically sp hybridization, which influences the shape and orientation of the sp hybrid orbitals involved in bonding.
Tip 3: Utilize molecular orbital theory to construct the molecular orbital diagram for CO, identifying the bonding and antibonding molecular orbitals.
Tip 4: Calculate the bond order using the formula: Bond Order = (Number of Bonding Electrons – Number of Antibonding Electrons) / 2.
Tip 5: Account for resonance structures, if applicable, as they can contribute to the overall bond order by delocalizing electrons.
Tip 6: Validate your calculated bond order by comparing it with experimental data or theoretical predictions from reliable sources.
Tip 7: Extend the concept of bond order to other molecules beyond CO, understanding how it relates to bond strength and molecular stability.
Tip 8: Utilize computational chemistry software or online tools to assist with complex bond order calculations, ensuring accuracy and efficiency.
Following these tips can improve the accuracy and efficiency of your bond order calculations for CO and provide a deeper understanding of its chemical bonding. In the concluding section, we will explore advanced applications of bond order in CO chemistry and its implications for various scientific disciplines.
Conclusion
Throughout this article, we have explored various aspects of “how to calculate bond order for co” and its significance in understanding the chemical behavior of carbon monoxide. Key points include the determination of atomic orbital overlap and hybridization, the construction of molecular orbital diagrams, and the application of bond order calculations to determine bond strength and molecular stability.
The ability to calculate bond order for CO provides valuable insights into its electronic structure and bonding characteristics. This understanding has practical applications in diverse fields such as catalysis, materials science, and atmospheric chemistry, where CO plays a crucial role. By accurately calculating bond order, scientists and researchers can design efficient catalysts, develop novel materials, and better understand environmental processes involving CO.
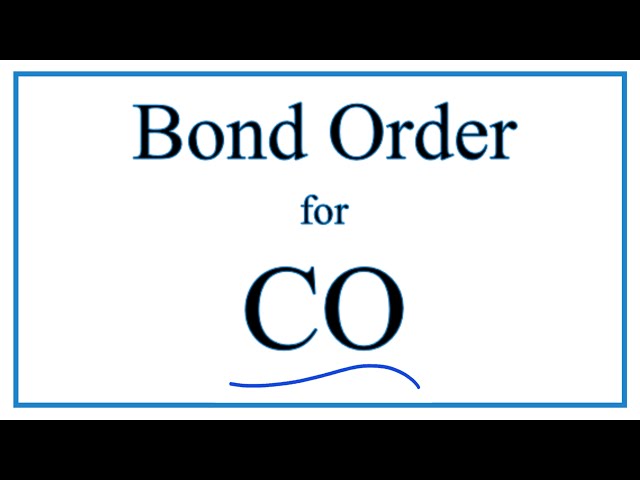