Bond order is a chemical concept that describes the strength and stability of a bond between two atoms. Determining bond order is essential in understanding molecular structure, chemical bonding, and predicting chemical properties. One key example is in the field of materials science, where understanding bond order helps in designing and optimizing advanced materials with tailored properties.
Calculating bond order provides crucial insights into the nature of chemical bonds. It has significant implications in various fields, including chemistry, materials science, and biochemistry. One notable historical development in this area was the introduction of quantum chemistry and the development of molecular orbital theory, which provided a more comprehensive understanding of bond order and its relationship to molecular properties.
In this article, we will delve into the details of calculating bond order, exploring the methods and techniques used to determine this important chemical parameter.
How to Calculate Bond Order Co
Calculating bond order is a fundamental aspect of understanding chemical bonding and molecular structure. It provides valuable insights into the strength, stability, and properties of chemical bonds. Key aspects to consider when calculating bond order include:
- Atomic orbitals
- Electron configuration
- Molecular orbital theory
- Bond length
- Bond energy
- Resonance
- Hybridization
- Delocalization
These aspects are interconnected and influence the overall bond order. For instance, the type of atomic orbitals involved in bonding determines the strength and shape of the molecular orbital formed. Bond length and bond energy are directly related to bond order, with shorter and stronger bonds having higher bond orders. Resonance and delocalization can lead to fractional bond orders, indicating the spread of bonding electrons over multiple atoms. Understanding these aspects enables chemists to accurately calculate bond order, which is crucial for predicting and explaining the behavior of molecules.
Atomic Orbitals
Atomic orbitals play a critical role in determining bond order, as they define the spatial distribution of electrons around atoms. The type of atomic orbitals involved in bonding, such as s, p, or d orbitals, influences the shape and strength of the resulting molecular orbital. For example, sigma () bonds are formed by the head-to-head overlap of atomic orbitals, while pi () bonds are formed by the lateral overlap of atomic orbitals. The number and type of atomic orbitals that overlap determine the bond order.
Understanding atomic orbitals is essential for accurately calculating bond order. By considering the atomic orbitals involved in bonding, chemists can predict the bond length, bond energy, and other properties of the molecule. This understanding is crucial in various fields, including materials science, where the properties of materials are directly related to the bond order of the constituent atoms.
In summary, atomic orbitals are fundamental to calculating bond order. The type and overlap of atomic orbitals determine the strength and characteristics of the formed bond. This understanding is vital for predicting molecular properties and designing materials with tailored functionalities.
Electron configuration
Electron configuration, which describes the distribution of electrons in atomic orbitals, plays a pivotal role in calculating bond order. The number and arrangement of electrons in the valence orbitals of atoms determine the type and strength of bonds they can form. For instance, atoms with unpaired electrons in their valence orbitals can participate in covalent bonding to achieve a stable electron configuration. The number of unpaired electrons and the orientation of the orbitals involved influence the bond order.
Understanding electron configuration is essential for accurate bond order calculations. By examining the electron configurations of the atoms involved in a bond, chemists can predict the type of bond that will form and its strength. This understanding is crucial in various fields, including materials science and inorganic chemistry, where the properties and behavior of materials are directly related to the bond order of the constituent atoms.
In summary, electron configuration is a critical component of calculating bond order. The distribution of electrons in atomic orbitals determines the bonding behavior of atoms, allowing chemists to predict and explain the properties of molecules and materials. This understanding is fundamental to many areas of chemistry and materials science.
Molecular Orbital Theory
Molecular orbital theory is a powerful tool for understanding and predicting the behavior of molecules. It provides a theoretical framework for calculating bond order, which is a key parameter in determining the strength and properties of chemical bonds.
- Atomic Orbitals: Molecular orbitals are formed by the linear combination of atomic orbitals. The type and symmetry of the atomic orbitals involved determine the shape and properties of the molecular orbital.
- Electron Configuration: The electron configuration of a molecule, which describes the distribution of electrons in molecular orbitals, influences the bond order. Molecules with unpaired electrons in degenerate molecular orbitals tend to have higher bond orders.
- Bonding and Antibonding Orbitals: Molecular orbitals can be classified as bonding or antibonding. Bonding orbitals have lower energy and promote bonding between atoms, while antibonding orbitals have higher energy and weaken bonds.
- Molecular Orbital Energy Diagrams: Molecular orbital energy diagrams show the relative energies of the molecular orbitals in a molecule. These diagrams can be used to predict the stability and reactivity of the molecule.
Molecular orbital theory provides a comprehensive framework for understanding and calculating bond order. By considering the interactions between atomic orbitals and the distribution of electrons in molecular orbitals, chemists can gain insights into the nature and strength of chemical bonds. This understanding is essential for predicting molecular properties, designing new materials, and understanding chemical reactions.
Bond length
Bond length is a critical component of calculating bond order, as it is inversely related to bond strength. A shorter bond length typically indicates a stronger bond and a higher bond order. This relationship arises from the fact that shorter bonds have greater overlap between atomic orbitals, leading to increased electron density in the bonding region. Conversely, longer bonds have weaker overlap and lower electron density, resulting in a lower bond order.
For instance, in the case of a carbon-carbon single bond, the bond length is approximately 1.54 angstroms, corresponding to a bond order of 1. In contrast, a carbon-carbon double bond has a shorter bond length of 1.34 angstroms and a higher bond order of 2. This difference in bond length reflects the increased overlap and electron density in the double bond compared to the single bond.
Understanding the relationship between bond length and bond order is essential for predicting the properties and reactivity of molecules. Shorter bonds with higher bond orders are generally stronger and less reactive, while longer bonds with lower bond orders are weaker and more reactive. This understanding finds practical applications in various fields, including materials science, where the strength and stability of chemical bonds are crucial for determining the properties of materials.
Bond energy
Bond energy, a measure of the strength of a chemical bond, plays a crucial role in determining bond order. It represents the amount of energy required to break a bond and is directly related to the stability of the molecule. A higher bond energy corresponds to a stronger bond and a higher bond order.
Bond energy is a critical component of calculating bond order because it reflects the strength of the overlap between atomic orbitals. Stronger overlap leads to lower bond energy and higher bond order. For instance, in the case of a carbon-carbon single bond, the bond energy is approximately 347 kilojoules per mole (kJ/mol), corresponding to a bond order of 1. In contrast, a carbon-carbon triple bond has a bond energy of 839 kJ/mol and a bond order of 3. This difference in bond energy highlights the stronger overlap and increased bond order in the triple bond.
Understanding the relationship between bond energy and bond order is essential for predicting the properties and reactivity of molecules. Compounds with stronger bonds (higher bond energies and bond orders) are generally more stable and less reactive, while compounds with weaker bonds are less stable and more reactive. This understanding finds applications in various fields, including materials science, where the strength and stability of chemical bonds are crucial for determining the properties of materials.
Resonance
In the realm of chemistry, resonance plays a pivotal role in understanding and calculating bond order. Resonance is a phenomenon that occurs when a molecule or ion can be represented by multiple valid Lewis structures, each contributing to the overall electronic structure of the species. This concept is particularly relevant in determining bond order, as it affects the distribution of electrons and the strength of bonds within the molecule.
Resonance affects bond order by delocalizing electrons across multiple atomic centers. When a molecule or ion has resonance structures, the electrons involved in the resonance are not confined to a single bond but are spread out over several bonds. This delocalization leads to a decrease in the bond order of individual bonds and an increase in the overall stability of the molecule.
A classic example of resonance is benzene, a six-membered ring of carbon atoms. Benzene has two resonance structures, each with alternating single and double bonds between the carbon atoms. The actual electronic structure of benzene is a hybrid of these two resonance structures, resulting in all carbon-carbon bonds having a bond order of 1.5. This delocalization of electrons contributes to the stability and unique chemical properties of benzene.
Understanding the concept of resonance is crucial for accurately calculating bond order and predicting the properties of molecules. By considering resonance structures and the delocalization of electrons, chemists can gain deeper insights into the electronic structure and bonding behavior of molecules. This understanding has practical applications in various fields, such as organic chemistry, materials science, and biochemistry.
Hybridization
In the context of calculating bond order, hybridization plays a crucial role in determining the electronic structure and bonding behavior of molecules. Hybridization involves the intermixing of atomic orbitals to form new hybrid orbitals with specific shapes and energies, which influences the geometry and properties of the molecule.
- sp Hybridization: Occurs when one s orbital and one p orbital combine to form two equivalent sp hybrid orbitals. These hybrid orbitals have a linear geometry and are commonly found in molecules with double bonds, such as carbon dioxide (CO2).
- sp2 Hybridization: Involves the combination of one s orbital and two p orbitals to form three equivalent sp2 hybrid orbitals. These hybrid orbitals have a trigonal planar geometry and are found in molecules with trigonal planar structures, such as ethylene (C2H4).
- sp3 Hybridization: Occurs when one s orbital and three p orbitals combine to form four equivalent sp3 hybrid orbitals. These hybrid orbitals have a tetrahedral geometry and are found in molecules with tetrahedral structures, such as methane (CH4).
- dsp3 Hybridization: Involves the combination of one d orbital, one s orbital, and three p orbitals to form five equivalent dsp3 hybrid orbitals. These hybrid orbitals have a trigonal bipyramidal geometry and are found in molecules with octahedral or square pyramidal structures, such as phosphorus pentachloride (PCl5).
Understanding hybridization is essential for accurate bond order calculations. By considering the hybridization of the atoms involved in a bond, chemists can determine the type and strength of the bond formed. This understanding has practical applications in various fields, including inorganic chemistry, organic chemistry, and materials science, where the properties and behavior of molecules and materials are directly related to the hybridization of the constituent atoms.
Delocalization
In chemistry, delocalization refers to the spreading out of electrons over multiple atoms or regions within a molecule or ion. It plays a crucial role in determining bond order and understanding the electronic structure of molecules. Delocalization affects bond order by altering the distribution of electrons involved in bonding, leading to fractional bond orders and unique chemical properties.
One prominent example of delocalization is in resonance structures. When a molecule or ion has multiple valid resonance structures, the electrons involved in resonance are not confined to a single bond but are spread out over several bonds. This delocalization lowers the bond order of individual bonds and increases the overall stability of the molecule. For instance, in benzene, the six carbon atoms form a ring with alternating single and double bonds. However, due to resonance, the electrons in the ring are delocalized, resulting in all carbon-carbon bonds having a bond order of 1.5.
Understanding delocalization is critical for accurately calculating bond order and predicting the properties of molecules. By considering the delocalization of electrons, chemists can gain insights into the electronic structure and bonding behavior of molecules. This understanding has practical applications in various fields, including organic chemistry, materials science, and biochemistry. For example, delocalization is essential for understanding the stability and reactivity of conjugated systems, which play a vital role in organic semiconductors and dyes.
Frequently Asked Questions on Calculating Bond Order
This section addresses common questions and misconceptions regarding the calculation of bond order. These FAQs aim to clarify key concepts and provide additional insights into the topic.
Question 1: What is bond order, and why is it important?
Answer: Bond order is a measure of the strength and stability of a chemical bond. It helps predict molecular properties and understand chemical reactions. A higher bond order indicates a stronger bond.
Question 2: How do I calculate bond order using the molecular orbital theory?
Answer: Molecular orbital theory involves examining the interactions between atomic orbitals to form molecular orbitals. The number of electrons in bonding molecular orbitals contributes to bond order.
Question 3: Can resonance affect bond order calculations?
Answer: Yes, resonance can delocalize electrons, leading to fractional bond orders. Resonance structures contribute to the overall electronic structure and stability of the molecule.
Question 4: How does hybridization impact bond order?
Answer: Hybridization involves the mixing of atomic orbitals to form hybrid orbitals with specific shapes. Different hybridization types influence the geometry and bond order of molecules.
Question 5: What is the relationship between bond order and bond length?
Answer: Bond order and bond length are inversely related. A shorter bond length typically indicates a higher bond order, reflecting stronger overlap between atomic orbitals.
Question 6: How can I use bond order to predict molecular properties?
Answer: Bond order provides insights into molecular stability, reactivity, and other properties. Higher bond orders generally correspond to more stable and less reactive molecules.
These FAQs provide a deeper understanding of the concepts surrounding bond order calculations. By addressing common questions, they clarify the role of various factors, such as molecular orbital theory, resonance, hybridization, and bond length, in determining bond order. This knowledge enables a more comprehensive analysis of chemical bonding and molecular behavior.
The next section will delve into advanced techniques for calculating bond order, exploring sophisticated methods used by computational chemists to tackle complex molecular systems.
Tips for Calculating Bond Order
Accurately calculating bond order is crucial for understanding chemical bonding and molecular properties. Here are five essential tips to enhance your bond order calculations:
Tip 1: Choose the appropriate method: Select the calculation method that best suits the complexity of the molecule and the desired accuracy.
Tip 2: Consider resonance and delocalization: Account for electron delocalization and resonance structures to obtain more accurate bond orders.
Tip 3: Utilize molecular orbital theory: Apply molecular orbital theory to analyze the interactions and energies of molecular orbitals, providing insights into bond order.
Tip 4: Understand hybridization: Determine the hybridization of atoms involved in bonding to correctly predict bond order and molecular geometry.
Tip 5: Relate bond order to bond properties: Establish the relationship between bond order and properties such as bond length, bond energy, and molecular stability.
By following these tips, you can refine your bond order calculations, leading to a deeper understanding of chemical bonding and molecular behavior.
The insights gained from accurate bond order calculations serve as a foundation for exploring advanced concepts in chemical bonding, such as molecular orbital theory and valence bond theory, discussed in the concluding section of this article.
Conclusion
Throughout this article, we have delved into the intricacies of calculating bond order, a fundamental concept in chemistry. We have explored various methods and techniques, emphasizing the importance of considering factors such as molecular orbital theory, resonance, hybridization, and bond properties.
Key points to remember include:
- Bond order provides valuable insights into the strength and stability of chemical bonds, influencing molecular properties and reactivity.
- Computational methods, such as molecular orbital theory, offer powerful tools for calculating bond order in complex molecules.
- Understanding bond order is crucial for predicting and manipulating molecular behavior, with applications in diverse fields such as materials science and drug design.
As we continue to unravel the complexities of chemical bonding, the accurate calculation of bond order remains a cornerstone of our understanding. It opens doors to further exploration of molecular structures, properties, and reactivity, paving the way for advancements in various scientific disciplines.
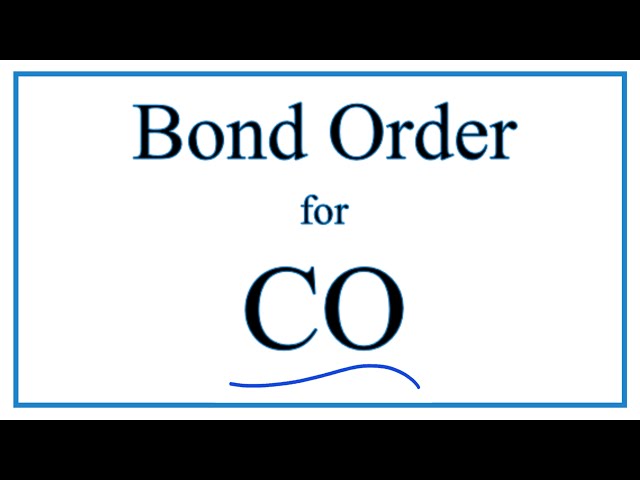